Nuclear energy : changing the balance of power
List of abbreviations and acronyms
Introduction
Key takeaways
Nuclear power today and tomorrow
Global trends
2. Advanced economies
Emerging and developing economies
Decarbonisation of the energy system
Integration and optimisation with renewables and other technologies
Decommissioning, lifetime extensions and replacements
Power markets, investments and support mechanisms
Other energy uses
Geopolitical implications
Manufacturing and technology export
Energy security, resilience and the impact of the Covid-19 crisis
Technology innovation
Medical radioisotopes
Conclusion
Summary
Nuclear power has been a major low-carbon source of electricity production for decades, ensuring affordable and reliable energy to millions around the world. Today, the nuclear industry is still very active, with 2018 being the record year of capacity additions in almost three decades, over 50 reactors under construction worldwide and many more planned. Innovation is being pursued on numerous fronts, from new generation types to small modular reactors, and from experimental reactors to nuclear fusion. The centre of gravity of nuclear deployment has shifted markedly in recent years from advanced to emerging and developing economies and is set to continue as they double their market share in global nuclear capacity over the next twenty years. Energy security, low-carbon electricity generation and reliability are crucial elements underpinning this deployment. However, nuclear power also has significant economic development and geopolitical implications. On the back of strong and consistent domestic deployment programmes, Russia is currently the largest exporter of nuclear technology, and China is set to become a major player as well. Advanced economies – once the major player and exporter – are now facing the ageing of their fleets, the evaluation of lifetime extensions and the need for new construction.
Nuclear is not without challenges: high upfront investment costs, means and costs of financing, delays in construction time, and strong opposition from population segments, in particular in some European countries that led to the immediate interruption of, or the gradual opt-out from nuclear programs. Solutions to the economic challenges exist, as Russia and China are clearly demonstrating. Europe has the largest nuclear fleet in the world, a fully integrated industrial supply chain and an important innovation ecosystem. For some regions, not pursuing or abandoning the nuclear option will make it significantly harder to reach decarbonisation goals in a timely and cost-effective manner. A carbon-free future requires all technologies to play their part, and nuclear can play a key role in these efforts, including for non-electric applications such as heat generation, hydrogen production and desalination. Policy makers should take action to ensure that the nuclear industry can play its part in contributing to the 2050 decarbonisation vision.
Marco Baroni,
Energy Expert, former Head of Power Generation Analysis in the World Energy Outlook team of the International Energy Agency and current energy consultant and lecturer at the Institut d’études politiques de Paris (Sciences Po).
List of abbreviations and acronyms
ARIS Advanced Reactor Information System (International Atomic Energy Agency, IAEA)
CCUS Carbon capture, utilisation and storage
CfD Contract for difference
DoE Department of Energy (United States)
DSM Demand side management
EC European Commission
EIA Energy Information Administration (United States)
FiT Feed-in tariff
GW Gigawatt
IAEA International Atomic Energy Agency
IEA International Energy Agency
IPCC Intergovernmental Panel on Climate Change
LCOE Levelised cost of electricity
LWR Light Water Reactor
MW Megawatt
NEI Nuclear Energy Institute (United States)
NRC Nuclear Regulatory Commission (United States)
PPA Power-purchasing agreements
PRIS Power Reactor Information System (International Atomic Energy Agency, IAEA)
PV Photovoltaic
PWR Pressurised Water Reactor
SDS Sustainable Development Scenario (International Energy Agency, IEA)
SMR Small Modular Reactor
VVER Water-Water energetic reactor
ZEC Zero Emission Credit
“Generation I” are the prototypes and power reactors that launched civil nuclear power in the 1950s and 1960s; “Generation II” reactors started in the late 1960s and account for the majority of reactors operating today; and “Generation III” reactors include significant fuel and major safety improvements (see section 3).
Following the definition of the International Monetary Fund (IMF), advanced economies include the members of the European Union, the United Kingdom, Switzerland, the United States, Canada, Japan and South Korea.
The Chernobyl nuclear accident took place in Ukraine on 26 April 1986 and started during a safety test of the reactor number It is considered the worst nuclear accident in the history of civil nuclear power.
The Fukushima Daiichi nuclear accident took place in Japan on 11 March 2011 following the Tōhoku earthquake and After the accident, Germany decided to shut down eight of its nuclear reactors.
The United Arab Emirates, Bangladesh, Belarus and The first of the four reactors being built in the United Arab Emirates – Barakah 1 – was connected to the grid in August 2020.
Policy makers have long been striving to provide accessible and affordable energy services in their countries. The growing importance of environmental issues and the potential harmful impact of certain energy uses has added to the concerns of ensuring that these services are also delivered sustainably. Today, two-thirds of the total greenhouse gases released globally are energy- related. This culminated in the Paris Agreement signed in 2015, with the aim of transforming the way that we produce and consume energy in a more sustainable manner. Energy systems have been continuously evolving since their inception, and new and further steps are needed to achieve the Paris goals.
Nuclear power is a technology that has ensured affordable and carbon-free electricity for decades in over thirty countries, with an estimated 60 Gt of CO2 saved globally over the past fifty years (see section II). Innovation is at the core of its prosperity: research and development actively continues, with new and safer reactors being developed and deployed around the world. Some 63 reactors came online worldwide over the last ten years (approximately one every two months on average) and there are 50 more currently under construction, the vast majority of which are of the safer and most modern type (called Generation III1).
The use of nuclear power has not only significant implications for energy security and the decarbonisation of electricity generation, but also for economic development, the energy industry, the resilience of energy systems (e.g. during the Covid-19 pandemic) and the geopolitical aspects of its deployment. Nuclear energy is particularly valuable in larger electricity systems, where reaching carbon reduction targets in a timely manner may be very difficult to achieve without this low-carbon energy technology, or made more challenging if its usage is reduced or phased out. This situation can be further exacerbated by the electrification of end-use sectors and the consequent increase of electricity demand, as required by many scenarios developed to reach the target of keeping global warming “well below 2°C”.
Two main nuclear energy trends can be observed today: advanced economies2 have a significant share of nuclear power in their mix with large but ageing nuclear fleets and must make urgent decisions on lifetime extensions and fleet renewal. Emerging and developing countries have low nuclear shares in the power mix and are looking to increase their fleets, with several of these countries having robust deployment plans, though they face financing challenges in some cases.
Advanced economies have been at the basis of nuclear power deployment – both domestically and abroad – which, in turn, sustained economic development, employment and industrial know-how. However, today Russia is the largest nuclear technology exporter and China is set to become a critical player soon. With many countries looking at deploying nuclear power, the technology is facing many opportunities to develop, yet several challenges need to be addressed and resolved beforehand.
Nuclear safety, waste disposal and the non-proliferation of nuclear weapons are primary public concerns in all countries. As proven by the Chernobyl disaster in 19863, accidents have large economic and societal consequences that respect no borders. Safety is a local, regional and international issue all at once, and public acceptance is key for the future deployment of nuclear power. The competence and independence of regulatory bodies is crucial, as well as the transparency and timeliness of information provided to the population. Advanced economies have long been at the forefront of this, notably in the United States, France, Canada and the Scandinavian countries.
The use of nuclear energy often polarises emotions, with some parts of population strongly opposing it while others support it. The nuclear accident of Fukushima Daiichi in 20114 further intensified these positions, with some people questioning whether nuclear energy can be considered a low-carbon and sustainable technology, while others firmly consider it as a viable option to address climate change.
These different approaches are reflected in the action (or inaction) of many governments, and have contributed to the slowdown in the development of nuclear energy in recent years. The spectrum of positions across countries is wide. Some countries have decided to accelerate the phase-out of nuclear power, following strong local opposition, such as Germany, while other European countries (Belgium, Switzerland and Spain) intend to opt out, although with different approaches and timings. Some countries decided to continue pursuing the nuclear option, in certain cases with a slowdown of starting new construction and changing towards newer and safer technology, such as in China. Some countries are keen to adopt nuclear technology, with four newcomers currently building their first reactors5, and others being at earlier stages of development. Some governments are supportive of nuclear, but face significant public opposition. Other countries face financial or market challenges, or have not yet implemented clear strategies or roadmaps. Overall, there is an equal, if not greater, number of countries that want to introduce nuclear power in the mix – mostly developing economies – with respect to the countries that want to stop using it.
The economics of new nuclear projects are an essential aspect as well. Cost and construction time overruns in several projects in Europe and North America have cast doubts on the economics of new reactors. These were the first Generation III reactors to be built in the respective countries, and further efforts will be needed to lower costs for further units. China, Russia and South Korea have been able to respect initial cost estimates and construction times in a much better fashion, mainly thanks to a continuous stream of construction of new reactors – a similar situation to that of France in the 1970s-80s.
Examining the historical evolution and the current situation of nuclear power, this paper focuses on fact-based analysis, leaving some policy considerations and recommendations in the conclusion, particularly related to the situation in Europe. This report looks at how innovation is essential for the continuous evolution of nuclear energy, how it can contribute to the decarbonisation of the energy sector, the geopolitical implications of the changing regional balance, and the challenges lying ahead for policy makers.
Key takeaways
Large power systems are defined here as those whose electricity generation is higher than 200 TWh per year.
- Nuclear power is still very active around the globe, but its centre of gravity for deployment has been From 1970-1999, three-quarters of new capacity worldwide was added in North America and Europe, and only 6% in Russia and China. From 2000-2019, this situation completely reversed, with almost two-thirds coming online in China and Russia and only 5% in North America and Europe. In 2018, new nuclear capacity added to power systems was at its highest levels since 1990, with 80% of additions in China and the remainder in Russia.
- China is set to surpass both Europe and the United States around 2030 in terms of installed nuclear power capacity, mainly with domestically developed China and Russia have stronger and better-defined nuclear power programs than most mature economies for future developments. In the mid-2030s, nuclear power capacity in emerging and developing countries will exceed that of advanced economies, even with replacements of existing reactors and deployment of new ones in the latter.
- Most large power systems use nuclear Three-quarters of large power-consuming countries6 use nuclear power in their mix, and account for almost 90% of global nuclear capacity. Power demand, energy security needs, technology innovation and its contribution to economic and industrial development, and therefore to economic sovereignty, are the main contributing factors. All major nuclear manufacturers are in the ten largest power systems.
- Nuclear power is an important decarbonisation option in the energy transition strategies of many countries. Reaching the Paris Agreement goals will require unprecedented efforts; renewable energy sources, energy efficiency measures, CCUS technology, and nuclear power all have roles to Limiting the range of choices will make it harder to attain goals and ultimately increase costs. Nuclear power is among the electricity-generating technologies that most contribute to the resilience of power systems and it demonstrated, especially in Europe, the ability of flexible operation – an essential factor in future power systems.
- Manufacturing for domestic and international markets has strong geopolitical Two-thirds of the international market adopted Russian and Chinese technology over the last ten years, and this share could grow with increasing Chinese manufacturing. The space to compete is vast, with more than 400 new reactors expected to be built worldwide over the next thirty years. Advanced economies need to make a strategic choice in this respect.
- Governments should evaluate and factor the risks and costs of losing industry know-how into their decisions. Innovation and research keeps being central for nuclear power: in small modular reactors, Generation IV reactors, nuclear fusion, experimental reactors (e.g. for medical use), but also for improving maintenance, fuel use and allowing for greater flexibility.
- The nuclear fleet is ageing: lifetime extensions and new builds are key decisions to be made by many countries in the near More than half of the nuclear fleet in advanced economies is set to retire in the next ten years if no action is taken. Lifetime extensions are the cheapest option for low-carbon dispatchable power generation in most countries, while new nuclear power plants have long lead times and some projects have shown high investment costs. Robust long-term planning and a constant stream of new builds are needed urgently to reduce costs, while providing more certainty to investors and visibility to the nuclear industry.
- Europe is at a Its nuclear fleet is the largest in the world and nuclear power is the largest electricity generating source, meeting one-quarter of its demand – as much as hydropower, wind and solar PV combined. Nuclear power has significant implications for energy security, the decarbonisation of energy systems, economic development, innovation, industry systems and geopolitical relationships, and can provide a significant contribution to the 2050 net-zero emissions target in the EU energy system.
Nuclear power today and tomorrow
The story of the power sector is that of a continuous change, innovating and refining the system and the elements that constitute it. The evolution of power generating technologies is at the core of this unceasing transformation, but transmission and distribution grids and effective management of end-use behaviour plays an important role now more than ever.
Initially based on hydropower and coal-fired generation primarily – that together accounted for approximately 80% of the global generation mix in 1950 – power systems have seen several major transformations over time. Oil-fired generation and the emergence of nuclear power were the two main transformations seen at the global level before the recent surge of wind and solar photovoltaic (PV) technologies (see figure below). Coal-fired electricity generation remained stable at around 40% of global power generation over the last fifty years, decreasing to around 35% in 2019 and even further in 2020 due to the Covid-19 crisis 7. Gas-fired generation saw a gradual growth in the global power share, with a strong increase in some countries and regions such as in the Middle East, North Africa, Russia or the United States (particularly over the last two decades and after the shale gas revolution).
Oil-fired generation increased sharply from around 9% to 25% of global power Generation In just ten years from 1963 to 1973. This rapid growth of oil in the global share of power generation was more than twice the increase in absolute percentage points compared with wind and solar PV, which collectively increased by around 7 percentage points over the past fifteen years. After the first oil shock of 1973, the share of oil-fired generation started to decline significantly in many countries, to currently reach less than 4%.
Global power generation by energy source, 1971-2018
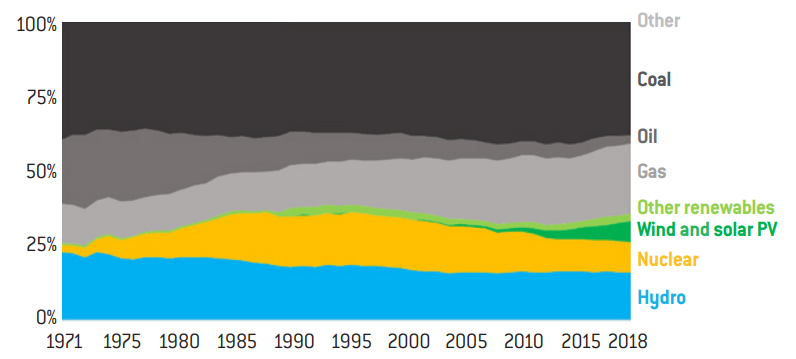
Copyright :
© Fondation pour l’innovation politique – December 2020
Source :
International Energy Agency (IEA), World Energy Balances, Paris, 2020.
With the decline of oil-fired generation, the rapid surge of a new energy source took hold: nuclear power increased from less than 2% in 1970 to almost 18% of the global mix in the beginning of the 1990s, mainly driven by its rapid expansion in advanced economies. The share of nuclear generation worldwide has declined in the XXIst century, due to stagnating additions and retirements in advanced economies, as well as the strong increase of electricity demand in emerging and developing countries, where the nuclear share is much lower. Its global share is now stable at around 10-11% since after the accident at Fukushima Daiichi in 2011.
In recent years, wind and solar PV registered rapid growth worldwide, on the back of strong support measures and declining costs, starting a third wave of transformation of the global power mix. Their last fifteen years of penetration in the global power mix is very similar to what was registered forty years prior by nuclear power (see figure below). Whether this increasing penetration into the power mix will follow in the footsteps of nuclear power, be lower, or exceed it, will critically hinge future investments and the measures that will be put in place after the Covid-19 crisis (see sections II.3 and III.2).
Share of nuclear, wind and solar PV in global electricity generation, 1963-2019
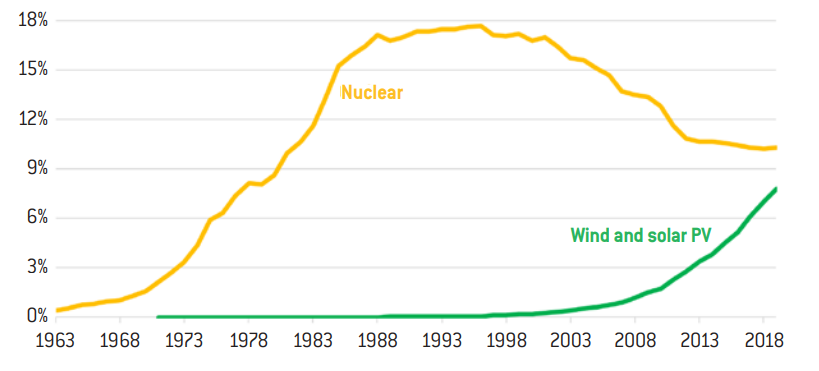
Copyright :
© Fondation pour l’innovation politique – December 2020
Source :
International Energy Agency (IEA), World Energy Balances, Paris, 2020 and author’s estimate over 1963-1970 based on historical nuclear capacity.
Global trends
a) Historical evolution of nuclear power
Nuclear power has been a success story of advanced economies for decades, but the centre of gravity has been – and still is – shifting over recent years. While most new nuclear plants constructions took place in advanced economies since its inception until the end of the last century, as of the beginning of the 2000s most of the additions took place in emerging and developing economies.
Small experimental reactors developed in the United States, the United Kingdom, France and Russia saw the birth of nuclear power during the 1950s. Ten more countries (Germany, Italy, Japan, Canada, Belgium, Sweden, Spain, the Netherlands, India and Switzerland) brought online nuclear reactors during the 1960s, and the decade closed with nuclear power barely accounting for 1% of global electricity generation. These early prototypes and the subsequent reactors that came online during the 1960s are often referred to as “Generation I”. By today’s standards, their generating capacity was quite small, averaging about 136 MW. More than 90% of them were deployed in advanced economies and have all subsequently been decommissioned.
The 1970s and 1980s saw the strongest growth of construction of nuclear reactors worldwide, with almost 400 reactors coming online during these two decades, and almost all of them of the “Generation II” type. The average size of these reactors was multiplied by a 7-8 factor with respect to the previous generation, and the total global installed capacity increased more than 20 times in twenty years. Similar to the previous generation, most of the additions took place in advanced economies, with 5 out of 6 plants being built in these countries (see table on the next page).
Two major accidents – the Three Mile Island accident in 1979 and the Chernobyl disaster in 1986 – intensified a slowdown in new construction starts, particularly in the United States and in Europe, that had already begun in the previous years due to several factors, including market saturation in certain countries (e.g. France), slower electricity demand growth in advanced economies and economic factors (e.g. financing issues). The slowdown continued during the 1990s, with Japan, France and South Korea accounting for almost two-thirds of the global capacity coming online over the decade.
Nuclear installed capacity and additions, 1950-2019
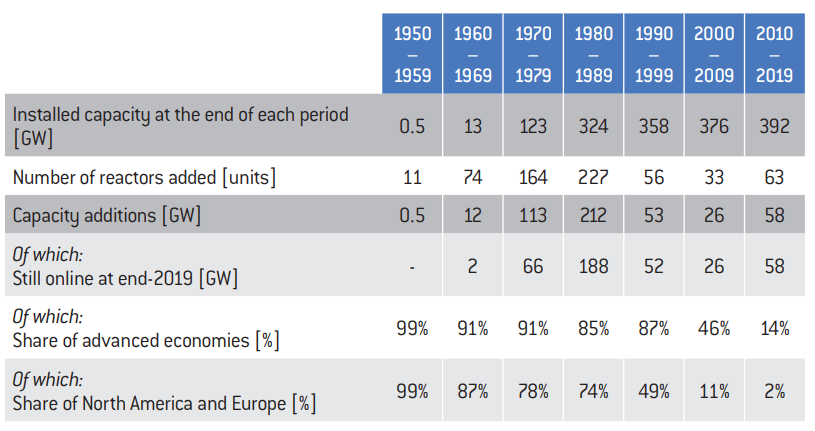
Copyright :
© Fondation pour l’innovation politique – December 2020
Source :
International Atomic Energy Agency (IAEA), Power Reactor Information System (PRIS) database, Vienna, 2020.
The 1990s and the first half of the 2000s saw the lowest level of new construction starts due to sluggish electricity demand growth in several advanced economies. Activity reaccelerated after 2005, particularly following a strong nuclear program in China that started the construction of 19 new reactors in just two years (2009-2010). The accident in Fukushima in March 2011 changed or slowed plans in several countries, including in China, where a clear shift from Generation II to Generation III was put in place. Despite the slowdown, 63 reactors started operation and an equal number started construction over the last ten years (2010-2019). Of these, 19 are already operational, while the others are expected to come online in the near-term.
Japan continued to deploy nuclear reactors for more than four decades until the Fukushima accident, after which no new reactors have started construction. Over the last fifteen years, South Korea started the construction of 10 new reactors, four of which followed the Fukushima accident and that are still under construction. In 2017, the country started a long-term phase-out policy, although some doubts exist as to whether this policy will remain in place after future elections.
Despite construction in Japan and South Korea limiting the drop of new builds in advanced economies, 80% of the new reactors that started construction in 2000-2019 were in emerging and developing countries (see figure above). China accounted for more than half of the new reactors within emerging and developing economies. Russia and India accounted for the bulk of the rest, but eight other emerging and developing countries also started new construction. Overall, almost two-thirds of the capacity that came online over the last 20 years (2000-2019) was added between China and Russia, and only 5% in North America and Europe, in stark contrast to the previous thirty years (1970-1999).
Number of reactors by start of construction and start of operation in advanced economies and emerging and developing economies, 1960-2019
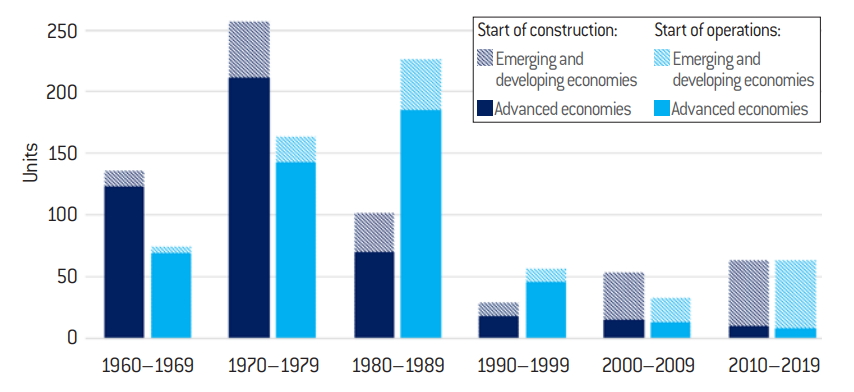
Copyright :
© Fondation pour l’innovation politique – December 2020
Source :
International Atomic Energy Agency (IAEA), Power Reactor Information System (PRIS) database, Vienna, 2020.
b) Nuclear power today
Nuclear power is still very active around the globe, despite the decision of several countries to abandon nuclear technology. In 2018, new nuclear capacity added to power systems worldwide was at its highest levels since 1990. All this capacity was added in between China (80%) and Russia (20%), compared to 1990 when more than 90% was added in advanced economies.
After a slowdown in 2019, the coming three years are expected to see similar levels of additions (on average) to those registered in 2018, though not breaking their record. This follows the status of the capacity currently under construction, with 53 reactors being built in 19 countries, and several approaching completion.
Advanced economies accounted for almost three-quarters of global nuclear capacity installed at the end of 2019 (see figure on the next page). Europe led the way, with 30% of global installed nuclear power, followed by the United States with 25%, but both with ageing nuclear fleets averaging 36 and 40 years respectively. Conversely, emerging and developing countries have young fleets and ambitious plans for further development. China has the youngest reactors (8 years on average for its 48 reactors) and the 3rd largest fleet in the world after the United States and France, having surpassed Russia in 2015 and Japan in 2018.
Today, about 95% of operating capacity is of the Generation II type, while the majority of the reactors under construction are of the Generation III type. Ongoing innovation has been a key characteristic in the evolution of nuclear technology throughout its development. Today, this research is focused on numerous possible new types, with several designs being explored for Generation IV, small modular reactors and ongoing research for nuclear fusion (see section III.3).
The different deployment of nuclear power between advanced economies and emerging and developing economies is reflected in its share in total electricity generation. The majority of advanced economies that use nuclear power have the share in the electricity mix between 20% and 40% (this was also the case for Japan before the Fukushima accident), with France surpassing 70%. Conversely, most emerging and developing economies have a nuclear share lower than 10%, with Russia and Ukraine being the most noticeable exceptions. While Russia produces about one-fifth of its electricity from nuclear power, China and India produce 4% and 3% respectively, leaving abundant space for deployment. In emerging and developing countries, the opportunity to displace fossil-fuel generation – coal-fired electricity generation in particular – is therefore quite immediate, both through the increase of generation from nuclear power and from renewable sources.
Note: European advanced economies include the countries in the European Union that have nuclear power plants plus the United Kingdom and Switzerland. Other advanced economies include Canada, Japan and South Korea.
Net installed capacity in 2019, number of units and average age by country/region
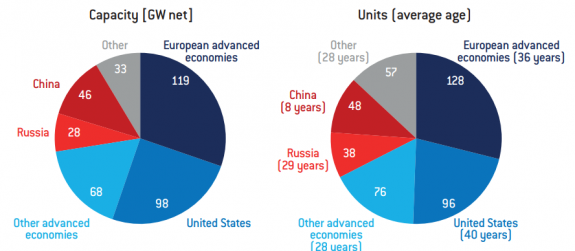
© Fondation pour l’innovation politique – December 2020
Source: International Atomic Energy Agency (IAEA), Power Reactor Information System (PRIS) database, Vienna, 2020.
The majority of large power systems have nuclear power in their electricity mix. There is a strong correlation between countries with high electricity consumption and the adoption of nuclear power in the generation supply mix. Globally, there are 21 countries that have total power generation higher than 200 TWh (see table on the next page). Three-quarters of these countries generate nuclear power. They represent half of the countries with nuclear power reactors, and they account for almost 90% of the global nuclear installed capacity. All major nuclear power manufacturers are in the ten largest power systems, further underlying the link between nuclear power deployment and industrial and economic development.
The smaller the power system, the fewer the countries with nuclear power: the share of countries using nuclear in their power mix decreases to just above one- third (36%) and one-fourth (25%) respectively for systems with total electricity generation from 100 to 200 TWh, and from 50 to 100 TWh. Only 4% of the countries whose electricity production is between 0 and 50 TWh per year are equipped with nuclear technology. While there may be several reasons why nuclear may be unsuitable for smaller power systems (e.g. financial cost, geography, geopolitical circumstances), a significant factor is that large power plants can be too large to handle in small power systems unless they are very well interconnected with neighbouring countries. On the other hand, in large power systems economies of scale can be achieved thanks to standardised and continuous construction. Small modular reactors could potentially change this situation, opening new markets to nuclear power (see section III.3.a).
Number of countries with nuclear power reactors, planning to add or phase out, by power system size, 2019
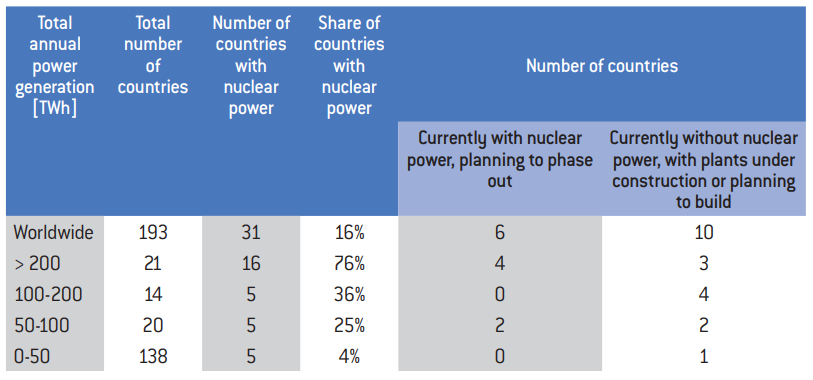
Copyright :
© Fondation pour l’innovation politique – December 2020
Source :
International Energy Agency (IEA), World Energy Balances, Paris, 2020.
Taiwan, province of China, as reported by the IMF.
More countries are planning to introduce nuclear power than those intending to phase it out. Following the Fukushima accident in Japan in 2011, concerns about the use of nuclear power led some countries to decide to phase it out. This goal is to be achieved either by early-retiring the nuclear reactors, or by letting them arrive to the end of their lifetime without replacing them. Currently, there are six countries with plans to abandon the use of nuclear power: Germany, Belgium, Taiwan9, Spain, Switzerland and South Korea, the first three by 2022-2025 and the latter three more in the long-term. South Korea is the only country within the group with reactors under construction.
Newcomer countries: under construction and most probable
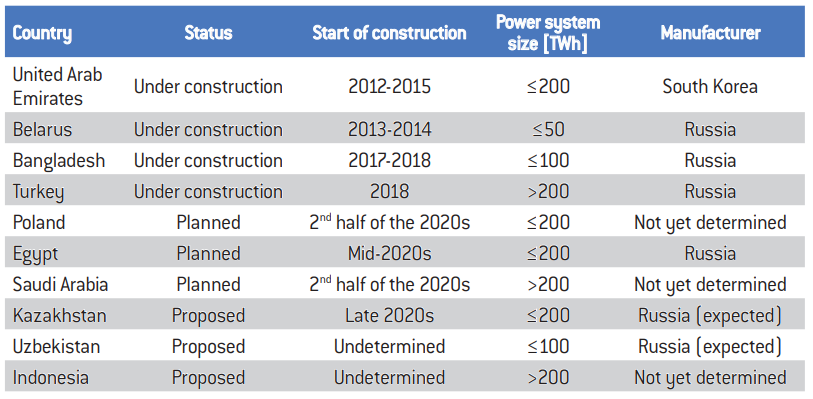
Copyright :
© Fondation pour l’innovation politique – December 2020
Source :
International Atomic Energy Agency (IAEA), Power Reactor Information System (PRIS) database, Vienna, 2020, IEA Statistics, newspapers articles.
Several countries without nuclear plants are envisaging to adopt this technology. Most of the countries that want to introduce nuclear power in their mix are emerging and developing economies with “medium-size” power systems (corresponding to the second and third group in the table on the previous page). Four countries are currently building new reactors – United Arab Emirates, Belarus, Bangladesh and Turkey – while several others are in different stages of planning (see table on the previous page).
2. Advanced economies
Nuclear Energy Agency NEA/OECD, Measuring Employment Generated by the Nuclear Power Sector, Paris, 2018.
Deloitte, Deloitte report Executive summary, 25 April 2019.
PricewaterhouseCoopers (PWC), Le poids socio-économique de l’électronucléaire en France, May 2011.
Cancellations of projects and a downturn of new start of construction already began before the TMI accident, due to a combination of factors, including financing The accident exacerbated this situation, with no new reactor starting to be built until recently, and with several delays for the reactors under construction at the time.
The EPR reactor, produced by Framatome, is a Generation III+ pressurised water reactor (PWR) with a typical size of 6 GW.
A power uprate represents the increase of the maximum thermal and electric output in an operating It can only take place after the United States’ Nuclear Regulatory Commission approval.
Advanced economies saw the highest level of deployment of nuclear power, also thanks to technology transfer within countries with high geopolitical coherence. The Light Water Reactor technology developed in the United States was transferred to Europe (e.g. Framatome in France), Japan, South Korea, and other countries, while the United Kingdom and Canada mainly continued to develop different types of technology until the 1980s.
Several of these countries developed a significant nuclear power manufacturing industry and significant industrial knowledge that contributed to economic development and to the creation of thousands of jobs during the construction of power plants, for the operation and maintenance of power plants throughout their lifetime, and for the entire fuel cycle. Direct, indirect and induced jobs are estimated in the order of more than 90.000 in South Korea10 and over a million in Europe11 of which 400.000 are in France12.
a) The United States
Nuclear power is an important part of the energy mix in the United States, but its future role will be contingent on policy choices. Until mid-last century, the power mix was primarily based on coal and hydropower. In 1950, these two sources respectively accounted for 46% and 32% of total power generation (see figure on the next page). Coal-fired generation met most of the electricity demand growth in the country until the early 2000s, maintaining or increasing its share for several decades, while hydropower’s share declined as the country was exhausting the potential for new sites, mainly due to environmental reasons.
From the first experimental reactors in the 1950s and the first limited inroads in the power mix during the 1960s, nuclear power saw its strongest growth in the 1969-1989 period, with a slowdown in 1979-1984 due to construction delays following the Three Mile Island (TMI) accident13. Electricity production kept increasing until the early 2000s and plateaued thereafter. The share of nuclear power in total U.S. generation has remained fairly constant over the last thirty years (1988-2019) – at around 20% – despite 9 reactors closing in the last seven years (2013-2019). The closure of this capacity, for a total of 6.8 GW (equivalent to about four EPR14 reactors), has been balanced over time by the uprates15 of operating reactors increasing total capacity in the country by 7.7 GW since the first uprate approval in 197716.
Share of power generation by selected source in the United States, 1949-2019
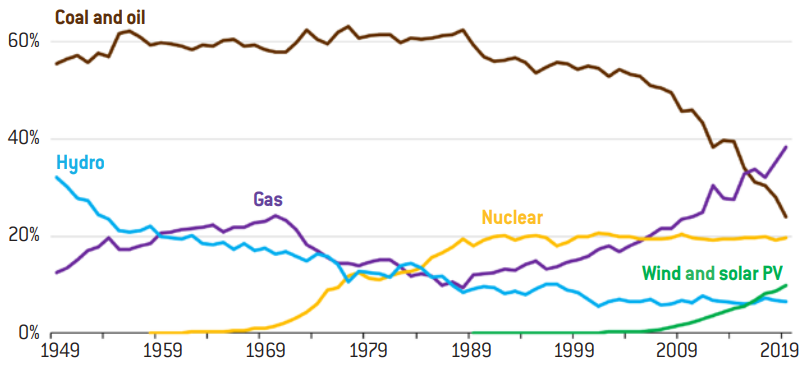
Copyright :
© Fondation pour l’innovation politique – December 2020
Source :
United States Energy Information Administration (EIA), retrieved in July 2020 and United States Energy Information Administration (EIA), Electric Power Monthly, retrieved in July 2020.
In this paper, “Europe” refers to the European countries that are part of advanced economies, as defined in the footnote on page It includes the countries in the European Union that have nuclear power plants or plan to have them, plus the United Kingdom and Switzerland.
In September 2020, Poland presented its updated energy policy for Offshore wind and nuclear power are two key pillars of this strategy. The first nuclear reactor is expected to be commissioned by 2033 and the following five units over the next ten years, for a total investment of around 35 billion euros.
Italy is the only European country among the larger power systems that does not have nuclear power.
Over the last ten years, coal-fired generation in the United States registered a very dramatic drop in both absolute and share terms, halving its share from about half to about one-quarter of the total. While nuclear and hydro remained virtually unchanged in share terms, two energy sources have substituted for coal-fired generation. Gas-fired generation compensated for two-thirds of the coal drop (thanks to low gas prices following the strong increase in the production of shale gas) and wind and solar PV took the remainder (mainly driven by support policies).
Currently, the United States has the largest nuclear fleet in the world (97 GW), spread over 29 out of 50 states. The vast majority of the plants have only one or two reactors each, with a maximum of three reactors per plant. This situation is quite different from all the other large nuclear producers (France, China, Russia, Japan, South Korea, India and Canada) that tend to have the majority of their nuclear plants with 4 to 8 reactors and a much less fragmented ownership with respect to the United States.
As of today, 70% of the fleet is concentrated in 12 states, with an average of 5 reactors per state, while the remaining 30% is in states with only 2 reactors on average. Most of the plants have been built by four manufacturers, with Westinghouse and General Electric being the major two. The lack of standardisation has often limited economies of scale and small individual plant sizes (22 plants have only 1 reactor and 32 plants have 2 reactors) can often lead to increased operating and maintenance costs and lengthy licensing processes for lifetime extensions. This can represent a hurdle, notably when competing in deregulated markets with the low gas prices that the country has been experiencing since the so-called “shale gas revolution”.
The United States started the construction of more than 90 reactors in the decade prior to the Three Mile Island accident. No new project was started thereafter for over thirty years until recently. At present, there are two new Generation III units under construction, while two other projects have been abandoned after Westinghouse filed for bankruptcy in March 2017.
While the future of new large reactors remains uncertain, with several projects put on hold or cancelled, the country has embarked on a research and development (R&D) programme on PWR-based small modular reactors as well as advanced reactors17. Several companies are investing in new designs and the first demonstration reactors are expected in the late 2020s. Similar efforts are being conducted in Canada, with which the United States is pursuing design standardisation.
The United States has not yet seen an overall decline of nuclear power generation, despite recent retirements. Almost all reactors have received permission to operate for sixty years, and four got the extension to eighty years (see section II.2). Nonetheless, several units beyond the nine that retired over the last seven years are foreseen to be decommissioned in the coming years (two more retired in January-October 2020) mainly due to adverse economic situations. A rapid decrease could be seen if urgent action is not taken to ensure that the proper conditions are in place for lifetime extensions and new projects, as well as ensuring that R&D and innovation continue to explore and secure new advanced technologies.
b) Europe18
In 2018, one-quarter of electricity generated in Europe came from nuclear power, more than any other single energy source and as much as hydropower, wind and solar PV combined. This share increases to 33% if only the countries which have nuclear power are accounted for. It is the largest low-carbon electricity generation source, providing a significant contribution to energy security and stability of power systems, to economic development and industry systems, with critical geopolitical relationships and implications. Nuclear power also contributes to a significant portion of electricity trade and to lower electricity prices in several European countries.
Altogether, the European advanced economies have the largest nuclear fleet in the world, and the second oldest on average after the United States. About half of the countries that use nuclear power in the world are in Europe. France alone accounts for more than half of the total European capacity and is the second largest fleet in the world by country. Similar to the United States, the strongest period of activity in Europe was from 1970-1980 when more than 120 reactors started construction. Three-quarters of Europe’s installed capacity operating today came online between 1978 and 1988.
After the Chernobyl disaster, the construction of new reactors almost halted in Europe, with only 7 reactors over the span of twenty years. Numerous reactors were closed before the end of their lifetime and Italy abandoned its nuclear programme. After the Fukushima accident, several reactors were retired early, mainly in Germany. Currently, four European countries have decided to phase-out the use of nuclear power: Germany and Belgium plan to close their reactors respectively by 2022 and 2025, while Spain and Switzerland plan to close theirs more in the long-term, by the mid-2030s. These four countries account for 24 GW, or 20% of total European nuclear capacity.
One-fifth of European electricity generation is produced today with nuclear power by countries that intend to keep – and in some cases, increase – this option. About half of European advanced economies use nuclear power today, or 15 out of 29 countries. Four of them intend to phase-it out, one to introduce it (Poland19), nine are adding or have plans to add new reactors and two have no firm plans (but have not ruled out the nuclear option – see table below). Currently, 6 reactors are being built in 4 countries, for a total capacity of 7.3 GW. The most notable exception among the countries with no nuclear power and no intention to include it in the power mix is Italy20, which, following the Chernobyl disaster, decided to close its nuclear plants in a referendum in 1987; the remaining 12 countries are smaller power systems, accounting for about 8.5% of European power generation altogether.
Installed capacity, average age and energy policies in place in European advanced economies
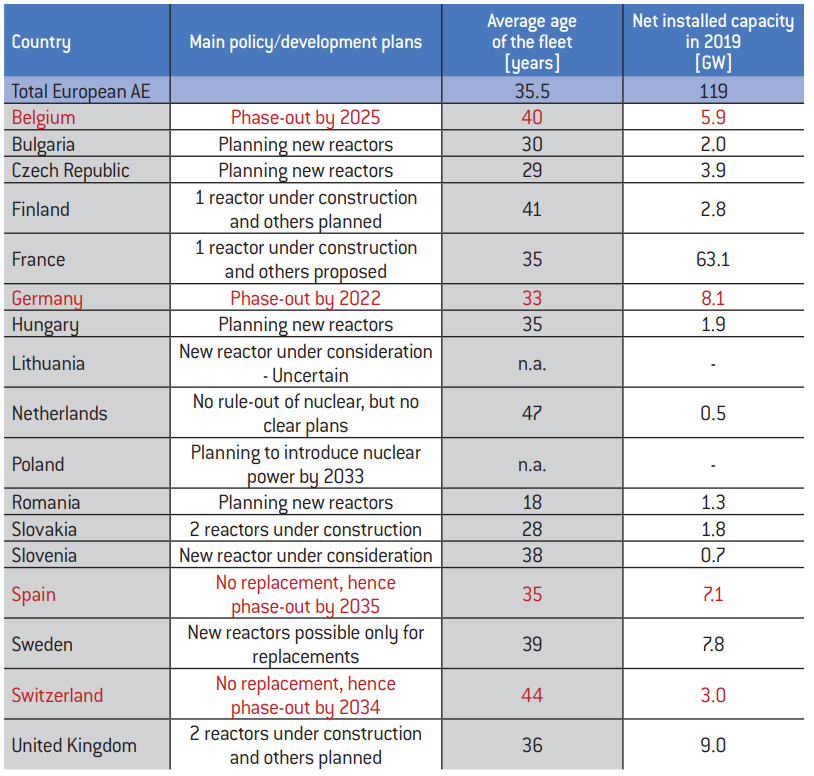
Copyright :
© Fondation pour l’innovation politique – December 2020
Source :
International Atomic Energy Agency (IAEA), Power Reactor Information System (PRIS) database, Vienna, 2020; several other sources.
Note: countries in red are those that are planning to phase-out their use of nuclear power.
International Energy Agency (IEA), Statistics, Paris, 2020.
United Kingdom Government, Department for Business, Energy and Industrial Strategy, Clean Growth Strategy: Leading the Way to a Low Carbon Future, London, April 2018.
European Commission (EC), A Clean Planet for All: A European strategic long-term vision for a prosperous, modern, competitive and climate neutral economy, 2018.
European Union (EU) Technical Expert Group on Sustainable Finance, Technical Report, Brussels, 2020.
The projections and analysis reflect solely the views of the The overall installed capacity presented for Europe would still fall short of the range presented in the scenarios of the European Commission, requiring either higher additions or additional lifetime extensions.
Seven countries that are building or planning to add new reactors are systems with total power generation of less than 100 TWh. Six out of seven are in Central and Eastern Europe, and another six out of seven currently have high shares from nuclear generation, typically in the range of 30-50%. European advanced economies are aiming at phasing out coal-fired generation. Several of the countries with a high share of nuclear power still significantly rely on coal-fired power generation and, as renewable potential is limited in some of them, nuclear remains an important option for decarbonisation.
A notable example in Europe is the United Kingdom, that has been able to push coal-fired generation almost entirely out of the mix, reducing it from 40% in 2012 to 5% in 2018, two-thirds thanks to increasing renewables (mainly wind and bioenergy) and one-third with gas-fired generation21. With one of the best wind offshore resources in the world, the United Kingdom seeks to meet low-carbon goals with a mix of renewables and nuclear power. Investing in innovation of all low-carbon technologies (including carbon capture, utilisation and storage – CCUS) is a key component of this strategy22. Nuclear power accounts for a stable 20% of generation in the country, but 14 out of the operating 15 reactors are set to be retired by 2030. There is an urgent need to replace them and a strong commitment from the UK government is required, but at this stage only two new plants are under construction, with several other projects on hold.
France has the largest fleet in Europe, and the highest share of nuclear power in the world. This was reached with a successful state-led programme, a strong industrial ecosystem (including fuel processing and reprocessing) and very competitive costs achieved by technological learning, standardisation and continuous construction (see figure in section II.3). The country plans to reduce the share of nuclear generation from more than 70% today to 50% by 2035, by retiring 14 of the oldest 900 MW units. One reactor is currently under construction, and the French government is also considering launching a program of 6 new EPR in order to start a partial renewal of the nuclear fleet in the early 2030s. This would temper the possible cliff-edge effect of the retirement of old reactors and support its nuclear industry, alongside the lifetime extension of several reactors (see section II.2). Any policy change in France will have a significant impact not only domestically (on energy security, decarbonisation and industry), but also on the overall nuclear trends in Europe.
Lifetime extensions and new construction are key decisions to be made in several European countries. Power systems require long-term planning, and European nuclear power reactors are set to face a rapid decline in the 2030s (see section II.2) unless clear actions are taken now to prepare robust energy strategy plans. Lifetime extensions are a low-cost option to keep low-carbon generation online and are essential to ensure that enough time is available to schedule and build replacement reactors.
Several scenarios are presented by the European Commission (EC) in its 2050 strategic long-term vision23, with nuclear power capacity in 2050 ranging from 100 to 120 GW, depending on the scenario. Considering the phase-out policy countries, the retirement of the operating reactors, the current plans in different countries and the possible long-term evolution, even to reach the low end of the EC vision, will require a significant increase in new construction throughout Europe.
At the end of 2019, the European Parliament and the Council reached a political agreement on the creation of a classification system for sustainable economic activities. The agreement was followed by a technical report in March 2020, with the related regulation coming into effect in the mid-2020s. This system, called EU Taxonomy, is based on six environmental objectives, sets performance thresholds and is intended to provide a tool to investors and lending institutions for the transition to a low-carbon, resilient and resource-efficient economy24. The inclusion or not of nuclear energy in the list of sustainable sources has been postponed and is subject to an assessment by the European Commission’s Joint Research Centre (JRC) to be published in 2021 (see section III.2).
In an assessment developed for the present report on possible lifetime extensions and the construction of new reactors following the plans of each European country, some 75 GW25 of new nuclear capacity would be added in Europe by 2050 (see figure on the next page). This amount would be split almost in half between France and the other ten European countries with nuclear power. In the analysis, 16 GW of new capacity are commissioned by 2035 (or 9 GW beyond the 7 GW already under construction), and a further 59 GW over 2036-2050. For France, this is equivalent to bringing 1-2 EPR reactors online by 2035 and a further 22-23 reactors by 2050. Despite being lower than the range envisaged in the EU’s long-term scenarios, it requires urgent action and long-term planning for the projects to come online in a timely manner.
Installed capacity in Europe, expected capacity remaining online and additions over 2020-2050
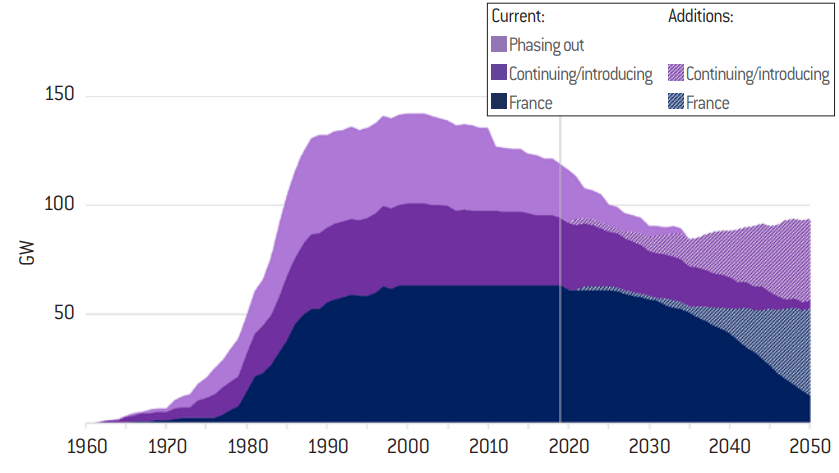
Copyright :
© Fondation pour l’innovation politique – December 2020
Source :
International Atomic Energy Agency (IAEA), Power Reactor Information System (PRIS) database, Vienna, 2020 for historical evolutions and for reactors under construction; author’s analysis for future evolutions.
These projects are often referred to as first of a kind (FOAK).
The amount of new capacity depends on a variety of factors, including the level of electricity demand, the number of lifetime extensions for existing reactors and the economics of new projects. The current projects26 in Finland and France have faced significant delays in construction and very high cost increases with respect to initial plans. Reducing these costs will be key to ensuring the future sustainability of new projects (see section II.3).
Emerging and developing economies
The share of emerging and developing countries in global nuclear installed capacity doubled over the last twenty years and is set to double again in the coming twenty. Nuclear power technology was primarily developed by advanced economies over the second half of the last century, but the centre of gravity of nuclear development and deployment has been shifting towards emerging and developing countries at the beginning of the 2000s. Indeed, over the last two decades, almost two-thirds of new global capacity came online in China and Russia, and a further 5% in India. With increasing additions in emerging and developing countries over the last two decades and retiring capacity (more than 50 GW) in advanced economies, the share of global installed capacity in emerging and developing countries doubled from 13% in 2000 to 27% in 2019.
The future deployment of new reactors faces several uncertainties in multiple countries, ranging from energy policies to economics, from access to financing to public acceptance. Some elements are, nonetheless, less uncertain than others. China has solid deployment plans (see section I.3.b), and so do Russia and India. Both Russia and China have strong ambitions to play a key role as exporters of nuclear technology. Many emerging and developing economies are looking at the nuclear power option for energy security and decarbonisation reasons. Industrial and economic development are also important factors, in particular for manufacturing countries.
In a scenario that considers a cautious implementation of the current plans of the different countries, accounting for several possible delays and the replacement of several retiring reactors (mainly in Europe and North America), one element emerges clearly: the decreasing role of advanced economies and the growing role of emerging and developing economies (see figure below) that reach 60% of global installed capacity by 2050, more than doubling today’s share. Pushed by the deployment of new reactors in China, emerging and developing economies will surpass advanced economies around 2035, with China and Russia alone surpassing them in the late 2040s. The level and speed of the deployment of new reactors in the coming years in China still presents uncertainties, though more on the upside than on the downside compared to those presented in this analysis.
Global nuclear installed capacity by country grouping, 1950-2050
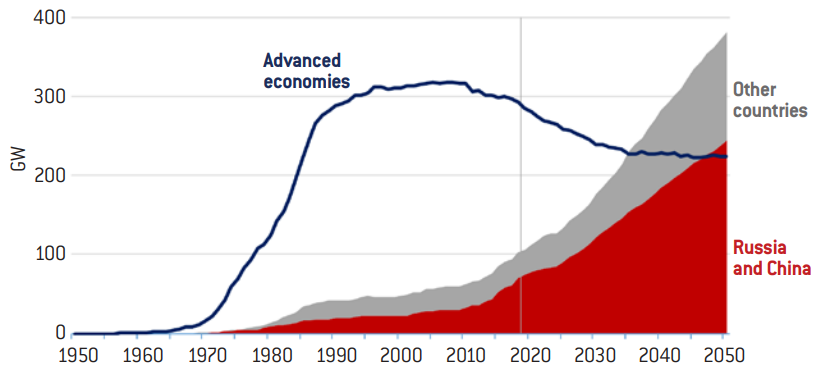
Copyright :
© Fondation pour l’innovation politique – December 2020
Source :
International Atomic Energy Agency (IAEA), Power Reactor Information System (PRIS) database, Vienna, 2020, numerous sources; author’s analysis.
a) Russia
Russia is currently the major exporter of nuclear power technology in the international market. The country has been one of the pioneers of nuclear power, deploying reactors since the 1950s. With 38 reactors in operation today, Russia is the fourth largest producer of electricity from nuclear in the world and meets almost 20% of its power demand with this energy source. The Russian Energy Strategy expects an increase in both nuclear installed capacity and share in power generation, although sluggish electricity demand is putting into question the exact amount and timing.
Half of the capacity in operation today came online during the decade 1979-1988, and one-quarter over the last ten years, increasing the total installed capacity by 30%. Eight reactors came online over the last five years, including a floating barge with two small modular reactors (SMR) of 35 MW each, for utilisation in remote locations. The country has three reactors currently under construction (plus another suspended one) and is on the verge of starting construction of four more units27. It is also building eleven reactors in six other countries, with further projects close to the start of construction in several other countries (see section III.1).
The large buildout programme – both domestic and international – took place mainly over the last ten years, with strong ambitions of the Russian state nuclear corporation Rosatom for the coming years and decades. This major overhaul of the nuclear industry followed a period of almost two decades after the Chernobyl disaster during which no new nuclear plant was started in the country. Several projects that were planned using the same technology as in Chernobyl (RMBK type) got cancelled, both inland and abroad.
The Russian nuclear industry is vertically integrated, with a strongly digitalised value chain and highly efficient manufacturing. Their flagship power plant is based on a Generation III type (of the VVER type, similar to the Western LWR technology), but Rosatom develops all types of reactors, including small modular reactors, floating and fast reactors. Research is very active, mostly on Generation IV type of reactors, as well as international cooperation, including the partnership to the ITER project on nuclear fusion (see section III.3.b).
Rosatom expects a growing part of its revenues to come from international projects. A decisive part of this strategy is the assistance in all steps of the project, including securing funding, building infrastructure, training employees, and securing long-term fuel supply chain.
b) China
China is set to surpass both Europe and the United States around 2030 in terms of nuclear power installed capacity, on the back of a robust deployment programme. China has a very young nuclear fleet, with an average age of only eight years per reactor. The country only saw its first nuclear capacity coming online in the 1990s, and its total capacity grew by a factor of 5 over the last ten years. In this period, China brought online 37 GW, or two-thirds of the total nuclear capacity additions globally.
Currently the 3rd largest fleet in the world after United States and France, it overtook Russia in 2015 and Japan in 2018. Nonetheless, nuclear only represents 4% of total power generation in China, leaving significant scope for further deployment. The country brought 28 reactors online over the last 6 years, equivalent to half of the entire nuclear fleet of France. This followed a very ambitious nuclear program launched in the 11th five-year plan, with a record start of construction of 19 new reactors in two years – for a total capacity of more than 20 GW – in a period when the country had a total peak of 9.2 GW.
Initially developed with French, Canadian and Russian technologies, nuclear power in China has been recently deploying both domestic and foreign technology recently, including United States’ Westinghouse AP-1000 and France’s EPR. The country has built considerable know-how right along the value chain (for engineering, manufacturing, and operation), with the exception of fuel reprocessing, for now.
The nuclear programme had a marked slowdown following the Fukushima accident, when the country decided to limit/halt the construction of Generation II and all inland projects, and to progressively move toward Generation III technology. By the end of 2019, China had half of the Generation III reactors operational in the world – including the first two French EPR and the first four United States’ AP-1000 that came online in the world – and another six under construction (out of 33 worldwide). Two of these reactors were started at the end of 2019, after a prolonged period without the start of any new construction, and a further two reactors were started in September-October 2020. Several observers expect this to be the re-start of the ambitious deployment programme, although its extent and pace are still unclear.
China developed a domestic Generation III design, the so-called Hualong-One, or HPR1000. Ten of these reactors are currently being built, of which eight are in China and two are in Pakistan. This is the flagship technology that China intends to export abroad. Four of the domestic projects are in an advanced state of completion and are expected to come online soon. Several more reactors are expected to start construction in the coming months and years, sustaining a continuous chain of construction and learning. The successful completion on-time and within budget for the first four domestic projects and the two in Pakistan are particularly crucial for China to demonstrate the viability of this technology for export purposes.
Decarbonisation of the energy system
Daniel Huppmann et , “IAMC 1.5°C Scenario Explorer and Data hosted by IIASA” (release 1.1), Integrated Assessment Modeling Consortium & International Institute for Applied Systems Analysis, 2018.
International Energy Agency (IEA), World Energy Outlook 2019, Paris, 2019.
International Energy Agency (IEA), World Energy Outlook 2014, Paris, 2014.
International Energy Agency (IEA), World Energy Outlook 2019, 2019, op. cit.
Nuclear power has been a key low-carbon option for decades and is set to play a significant role in the decarbonisation strategies of many countries. Globally, it is the second most important low-carbon electricity-generating source after hydropower, currently accounting for 16% of global power generation (with respect to 10% for nuclear power). In advanced economies, the share of nuclear in total power generation is twice as much, at a level around 40% higher than hydropower.
Today, the power sector is the largest global source of energy-related CO2 emissions. In addition, in order to reach the goals of the Paris Agreement, a significant electrification of end-uses can be expected, bringing an ever-growing role for this energy source. Therefore, the decarbonisation of the existing power sector and ensuring that low-carbon options are deployed to meet new demand growth will play a pivotal role in any future decarbonisation strategy. All low-carbon technologies are needed to achieve the targets in a timely manner. A mix of energy efficiency measures, renewable energy sources, nuclear energy and CCUS technology will be needed, as shown in the scenarios of the Intergovernmental Panel on Climate Change (IPCC) 28, the International Energy Agency29, and several other institutions.
From 1970 to today, nuclear power saved an estimated 60 Gt of CO2 globally30, equivalent to 60 times the emissions of the current power sector in the European Union. Limiting the future range of technology choices will make it harder and costlier to achieve decarbonisation goals. In 2018 in Europe, nuclear power generation was 60% higher than the generation from wind and solar PV combined. Installed capacity of solar PV surpassed that of nuclear in 2019, but its electricity generation was one-sixth of that of nuclear power. Replacing today’s nuclear power generation in the region would require a major effort, with no gains in CO2 emission reductions.
Overall, in Europe, replacing the current nuclear electricity production would require about 500 GW of wind and solar PV capacity, compared to the 300 GW currently installed. This amount is very similar to the 500 GW of wind and solar PV capacity increase by 2040 in the IEA’s Sustainable Development Scenario31. Therefore, to replace current nuclear generation, we would have to double the efforts deemed necessary to reach Europe’s decarbonisation goals in this ambitious scenario, reaching a total wind and solar PV installed capacity four times larger than today’s. This would significantly raise the hurdle of decarbonisation by 2050, including the associated grid development and its acceptability. Moreover, the time available for decarbonisation is a crucial element, as the “envelope” of total emissions is limited, and postponing CO2 reductions will make it more difficult to reach these goals.
Nuclear power can play a significant role, especially in large electrical systems. The countries that currently use nuclear power or that are building new plants can be broadly divided into 3 groups (see figures on the following pages):
- Countries with large hydropower shares in total power generation, where nuclear complements it to reach very low emissions per unit of power produced;
- Countries with low nuclear shares (0-10%), where the vast majority are emerging and developing economies, and emissions are typically in the 400- 800 gCO2/kWh range, with the largest coal and oil consumers being in the higher part of this range.
- Countries with higher nuclear shares, where the vast majority are advanced economies, and emissions are typically in the 250-500 gCO2/kWh range.
Three main trends can be highlighted: first, about 60% of the largest power systems (that generate more than 100 TWh) use nuclear in their power mix (see the table on page 20). Two-thirds of these countries have nuclear plants currently under construction. Second, among the power systems with low nuclear shares, two are going to phase-out nuclear and four “newcomers” are building nine reactors. The majority of countries in this group are large consumers and intend to increase their nuclear share, with China and India taking the lead. Third, among the systems with higher nuclear shares, three countries have plans to phase-out nuclear power in the long-term, while most of the others have plans to build new reactors.
About half of the countries with higher nuclear shares are smaller power systems with less than 100 TWh of electricity production. Of these ten countries, nine have a share of nuclear in total power generation in the range of 30-55%. The majority of these countries are in Central and Eastern Europe, where renewable resources are more limited and reliance on coal-fired generation is still strong. Nuclear power is a very important option for further decarbonisation and some countries expressed their disappointment in not including nuclear energy in the European Green Deal package.
CO2 emissions intensity and share of nuclear power in total electricity generation in power systems with nuclear power and with nuclear plants under construction, 2018
a) Power systems with nuclear power or currently building plants according to their CO2 emission intensity and the share of nuclear power in total electricity production
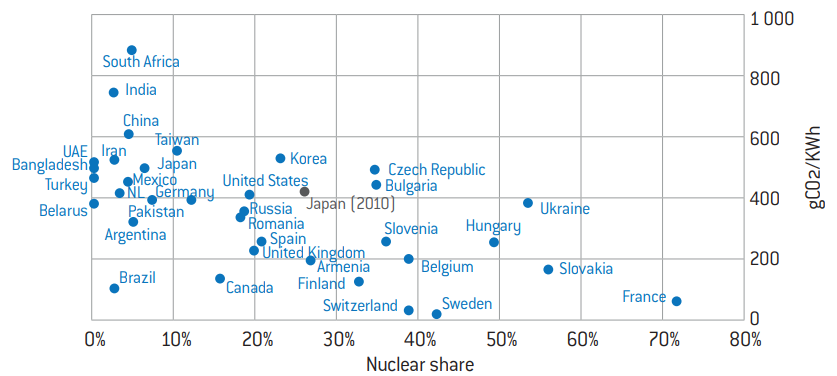
Copyright :
© Fondation pour l’innovation politique – December 2020
Note: “Japan (2010)” shows the situation before the Fukushima accident. Currently, 9 of the 33 operable reactors have restarted, with several others pending approval. The Fifth Energy Basic Plan targets a nuclear share of 20%-22% in total power generation by 2030. UAE means United Arab Emirates and NL means the Netherlands.
b) Power systems with nuclear power, according to their level of economic development
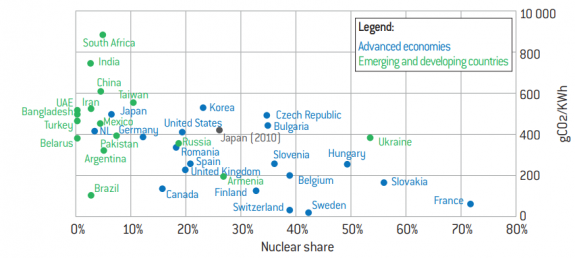
© Fondation pour l’innovation politique – December 2020
Source: International Energy Agency (IEA), World Energy Balances, Paris, 2020 and International Energy Agency (IEA), IEA Emissions factors, Paris, 2020.
c) Power systems with nuclear power, according to their nuclear power plant construction plans
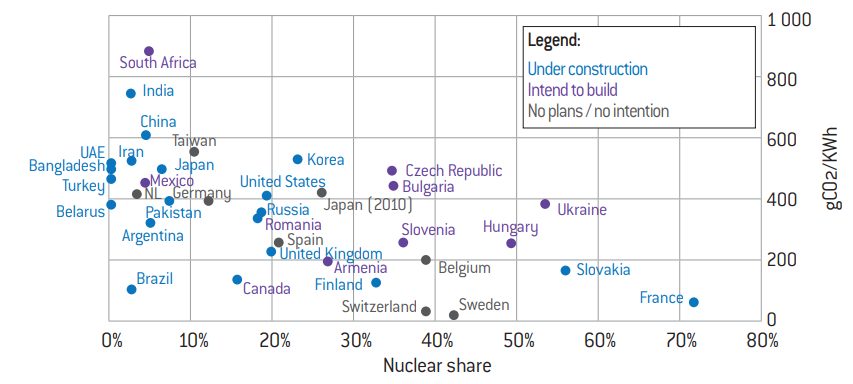
Copyright :
© Fondation pour l’innovation politique – December 2020
Note: “Japan (2010)” shows the situation before the Fukushima accident. Currently, 9 of the 33 operable reactors have restarted, with several others pending approval. The Fifth Energy Basic Plan targets a nuclear share of 20%-22% in total power generation by 2030. UAE means United Arab Emirates and NL means the Netherlands.
d) Overall view of power systems with nuclear power
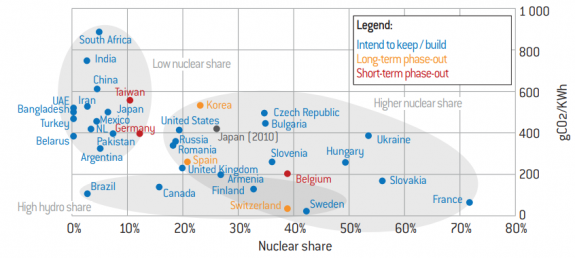
© Fondation pour l’innovation politique – December 2020
Source: International Energy Agency (IEA), World Energy Balances, Paris, 2020 and International Energy Agency (IEA), IEA Emissions factors, Paris, 2020.
International Energy Agency (IEA), World Energy Outlook 2019, 2019, op. cit.
France has a unique situation: with the highest nuclear power share of electricity generation in the world and a significant share of renewables, its emissions per unit of electricity generated are among the lowest in the world, at around 50-60 gCO2/kWh. It is the fifth lowest among advanced economies after Iceland, Norway, Sweden and Switzerland – all of which are large hydro producers – and is the lowest among the largest 20 power systems. Improving, or even maintaining this position while decreasing their nuclear share will be challenging.
Reaching the Paris Agreement goals requires a substantial reduction of the global CO2 emission intensity of the power sector, from around 480 gCO2/kWh in 2018 to 80 gCO2/kWh32 in 2040, and decreasing further in subsequent years. Only a few countries have a comparable level of emissions today, most of them with significant hydropower resources.
Increase in electricity generation by low-carbon technology between the Stated Policies and the Sustainable Development Scenarios of the IEA over 2018-2040
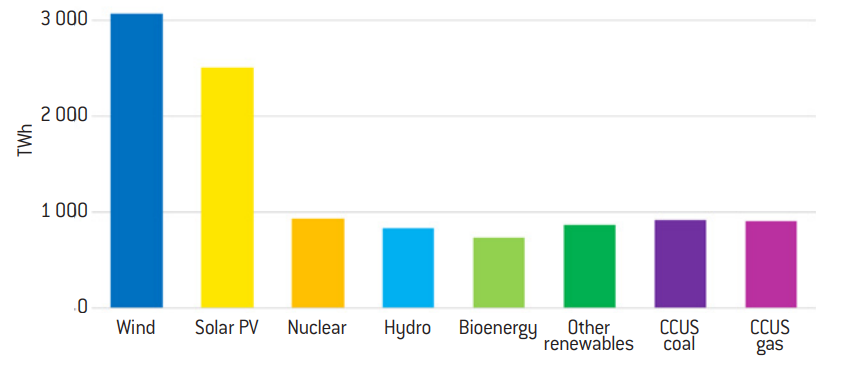
Copyright :
© Fondation pour l’innovation politique – December 2020
Source :
based on International Energy Agency (IEA), World Energy Outlook 2019, 2019, op. cit.
Nuclear power plays a significant role in most of the IPCC decarbonisation scenarios, though with different shares. Globally, it is the third largest contributor (see figure on the previous page) – after wind and solar PV – of the increase in low-carbon electricity generation between the Stated Policies and the Sustainable Development Scenarios (SDS) presented by the International Energy Agency33. Similar increases come from hydropower, CCUS with coal and CCUS with gas.
In the Sustainable Development Scenario, nuclear provides 11% of global electricity generation, or 13% of total low-carbon power in 2040. The share increases significantly in China and Russia, and generally across all emerging and developing economies, following today’s trends. By 2040, nuclear power in Europe will account for more than 1 kWh out of 6 kWh generated. This share doubles to around 40%34 when only the eleven countries with nuclear are accounted for.
Integration and optimisation with renewables and other technologies
The flexibility of power plants, and in general of power systems, relates to the ability to quickly change their generation (and the minimum amount that can be sustained before closing the plant) according to the variations of In future power systems, flexible operations will also be needed to accommodate the variability of generation of wind and solar PV technologies.
Nuclear Energy Agency NEA/OECD, The Costs of Decarbonisation: System Costs with High Shares of Nuclear and Renewables, Paris, 2019.
Nuclear Energy Agency NEA/OECD, Nuclear Energy and System Effects in Low-carbon Electricity Systems, Paris, 2012.
Paul Joskow, “Comparing the Costs of Intermittent and Dispatchable Electricity Generating Technologies” American Economic Review, 101 (3): 238-41, 2011.
Lion Hirth, “The Market Value of Variable Renewables”, Energy Policy 38, 218-236, 2013.
International Energy Agency (IEA), World Energy Outlook 2018, Paris, 2018.
United States Energy Information Administration (EIA), Levelized Cost and Levelized Avoided Cost of New Generation Resources in the Annual Energy Outlook 2019, 2019.
Clean Energy Ministerial (CEM), Flexible Nuclear Energy for Clean Energy Systems, 2020.
Jesse Jenkins et al., “The benefits of nuclear flexibility in power system operations with renewable energy”, Applied Energy, Vol. 222, pp. 872-884, 2018.
International Energy Agency (IEA), The Future of Hydrogen, Paris, 2019.
Deutsche Energie-Agentur (DENA), Integrated Energy Impulses to shape the energy system up to 2050, Berlin, 2018.
Reaching the energy transition goals requires tapping into all technologies. Without nuclear energy, it would be more difficult and costly. Renewable energy sources, nuclear power, and carbon capture, utilisation and storage (CCUS) are all key energy sources and technologies to decarbonise the electricity mix. Comprehensive planning needs to be put in place to ensure the coherency of policies to deploy these technologies, coupled with energy efficiency measures – the other major pillar of energy transition.
The optimal mix of low-carbon technology options needs to be carefully evaluated and identified based on potentials, economics, energy security and flexibility35 considerations for each country and balancing area36. Abandoning technology options – such as nuclear or CCUS – with a priori considerations is set to increase costs: some 1.6 trillion US dollars of additional investments would be needed from 2019 to 2040 if no lifetime extensions and new nuclear projects took place in advanced economies37.
In addition to replacing fossil fuels with low-carbon sources, substituting existing forms of low-carbon generation with alternative forms of low-carbon generation increases the challenge to achieve decarbonisation goals leading to additional efforts and costs. In Germany, half of the increase in renewable generation between 2005 and 2018 compensated for the decrease of nuclear power generation. The decrease in nuclear generation was twice the increase in electricity generation from solar PV, despite Germany having the largest solar PV deployment in Europe. Replacing the electricity production of the last six reactors scheduled to be decommissioned by 2022 will require the equivalent of an additional 75 GW of solar PV, or 1.5 times today’s installed capacity in Germany (around 50 GW).
The evaluation of the optimal power mix is a complex matter, as it needs to take many variables into account. Estimating the competitiveness of different technologies requires consideration of the overall system costs38, including balancing costs, adequacy costs, grid costs and the cost of integration measures. An indicator that is often used to compare technologies is the Levelised Cost of Electricity (LCOE). This indicator, though, provides only an approximation of costs, with no consideration for the value of the electricity produced and is therefore a flawed indicator to evaluate competitiveness39. As wind and solar PV have decreasing value of the electricity generated with increasing levels of penetration in the mix40, additional indicators are being developed to allow for a better comparison, such as the Value-Adjusted LCOE41 or the Levelised Avoided Cost of Electricity42.
The transformation of the power systems is going to require a profound change in the power mix, and several important modifications to the way in which we produce and consume electricity. Wind and solar PV are set to see their roles increase significantly. In order to accommodate their variability, the call on all flexibility options – both on the supply and the demand side – is going to increase too. The main integration options include the flexibility of dispatchable power plants, the increase of transmission and distribution grids, energy storage (e.g. pumped hydropower storage or batteries) and demand side management (DSM).
Nuclear power can provide a great degree of flexibility, if the appropriate design of the reactors is in place, therefore complementing wind and solar PV generation well43. Nuclear is often considered to be an inflexible technology, but examples of the French and German operations and the flexibility seen during the Covid-19 pandemic show what can be achieved with nuclear reactors (see section III.2). A study conducted in the United States44 shows that the flexible operation of nuclear plants, despite reducing output, can increase the revenues of theexistingplants.Additionalinvestmentstoallowsuchoperationsmaybeneeded, depending on the type of nuclear technology, to avoid additional wear and tear. As this type of operation will become crucial in future power systems, providing appropriate remuneration to the power plants providing this service is going to be essential (see section II.3).
The increasing number of distributed generation sources will also change the way in which grids are operated, both for transmission and for distribution networks. The expansion of power grids might pose challenges of public acceptance, with similar and sometime stronger difficulties than the siting of nuclear and CCUS power plants and land use for renewable sources.
The decarbonisation of the last few percentage points of total electricity generation and of some final sectors can prove to be difficult and costly to achieve. Nuclear can provide a significant contribution to hydrogen production in a stable and reliable way, although several studies still show that hydrogen production is expected to be relatively expensive45 (see section II.4).
The level of electrification of final sectors and of the production of hydrogen varies considerably among different studies and scenarios and directly impacts the level of deployment of wind and solar PV technologies, nuclear power, and other technologies. A recent study by the Deutsche Energie Agentur46 looks at the decarbonisation of the energy sector with two possible target levels and four scenarios, through the exclusive use of renewable energy sources. As renewable potentials reach their limits without the use of nuclear energy or of CCUS technology, the growing levels of decarbonisation ambitions bring growing amounts of energy imports in Germany from EU and non-EU countries.
Decommissioning, lifetime extensions and replacements
Including 5 reactors in Japan that have not produced electricity since 2011 and one reactor each in South Korea and Taiwan that have not produced electricity since 2017.
The global fleet is ageing rapidly, requiring urgent decision-making regarding lifetime extensions and replacements. The current average of the global nuclear fleet is 30 years, increasing to 37 on average in North America and Europe. A large majority of the reactors operating today is of the Generation II type (almost 95% of the total) and were initially designed for a lifetime of 35-40 years.
The initial foreseen lifetime can be extended to 60 years and in some cases even beyond, if the proper conditions are in place and significant investments to improve safety and replace necessary parts are undertaken. Examples are the ongoing refurbishment plan in Canada or the “Grand Carénage” project in France. Several other countries have either put plans in place or are in the midst of planning similar extensions. The vast majority of the reactors in the United States has received the licence to operate for 60 years and a few of them recently received approval for 80 years of operation, with several others considering applying.
Despite the extension having already been approved, in the United States low gas prices make it hard for nuclear plants to recoup costs, thus several plants faced early closures in recent years. As with investments in capacity additions, political uncertainty also impacts investment decisions for lifetime extensions. Other economic factors can outweigh the profitability of the additional investment requirements: additional taxes and low wholesale prices can render the additional investment unattractive.
Lifetime extensions are the cheapest low-carbon, dispatchable generating option in most countries, maintaining carbon-free power generation in the mix and reducing potential calls on fossil-fuel plants. Additionally, they are critical in allowing long-term planning to be put in place. New reactors, in fact, take several years of planning and construction, and robust plans need to be put in place if replacement – or expansions – of the fleet are envisaged.
Adverse economics and ageing of the fleet point towards a possible wave of the decommissioning of nuclear reactors at unprecedented levels in the coming years. In 2019, 13 units were retired worldwide47, 85% of which were in advanced economies. This level had been reached only twice in history, with peaks in retirements immediately following the Chernobyl and Fukushima accidents. Globally, 85 units (almost 20% of a total of 443 units around the world) are more than 40 years old. Of these, 70 units (or 23% of a total of 300 units) are in advanced economies.
With no or limited extension, more than half of the global fleet is at risk of closure over the next decade (see figure below). This is equivalent to 25% more than all the retired reactors worldwide in history, with significant consequences on energy security, CO2 emissions and the ability of the industry to decommission so many plants at the same time.
Even with lifetime extensions, over the next thirty years, around 270 reactors will be decommissioned, almost three-fourths of which are in advanced economies. More than 60 reactors are set to be retired in the next six years alone. With lifetime extensions, about one-quarter of the reactors in advanced economies are set to be retired over the next decade (2020-2030), compared with more than half in the case of no extensions.
Note: The “no lifetime extension” scenario results in a 42 year lifetime on average globally, excluding the United States, and 52 years in the United States. The “with lifetime extension” scenario results in an additional 10 years of lifetime on average globally, and an additional 15 years in France and the United States.
Decommissioning of nuclear reactors worldwide, 1960-2050
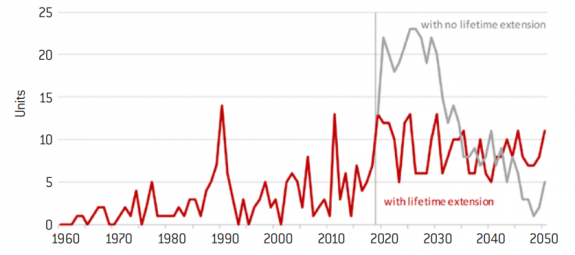
© Fondation pour l’innovation politique – December 2020
Source: International Atomic Energy Agency (IAEA), Power Reactor Information System (PRIS) database, Vienna, 2020 and the author’s analysis.
Power markets, investments and support mechanisms
See for example: Paul Joskow, Challenges for Wholesale Electricity Markets with Intermittent Renewable Generation at Scale: The U.S. Experience, MIT CEEPR Working Paper, December 2018.
Manfred Hafner et , The Geopolitics of the Global Energy Transition, Policy and Regulation of Energy Transition chapter, Springer Nature, Volume 73, 2020.
International Energy Agency (IEA), Nuclear Power in a Clean Energy System, 2019, op. cit.
Massachusetts Institute of Technology (MIT), The Future of Nuclear Energy in a Carbon-Constrained World, 2018.
Low-carbon technologies are capital intensive, and most of them require support measures. Nuclear power is no exception. Liberalised electricity markets have been introduced in a period that was dominated by fossil-fuel generation, though with a significant portion of hydropower and nuclear power. The objective was to optimise the power plants dispatching in the short- term and provide long-term signals for investments in new assets.
The aim of steering away from fossil-fuel electricity generation is changing this model. The introduction of large shares of wind and solar PV in the market typically depresses electricity prices, making it difficult for most technologies (including wind and solar PV themselves) to invest in new capacities without some form of support. Additional revenues may be made available through supplementary market mechanisms for dispatchable firm capacity that is available at peak times and can provide flexibility to the system. Nonetheless, the existence of power markets for long-term signals is put into question in several studies48. Support measures are especially needed for low-carbon technologies, as investments based purely on market forces have not proven to be viable.
Wind onshore, offshore and solar PV technologies installed capacities have increased strongly over the last fifteen years, on the backs of support measures and falling costs due to economies of scale. Support measures have been changing and evolving throughout time, as policy makers were looking for more sophisticated and better forms of support. They include feed-in-tariffs (FiTs), premiums, tax exemptions or credits, contract for difference (CfD), zero emission credits (ZEC), and long-term power purchase agreements (PPAs), with or without auctions to award them49. Initially designed for supporting renewable energy sources, they are now also used for other low-carbon technologies (e.g. CfD for nuclear power in the United Kingdom) and in some cases also for fossil-fuel capacity to ensure that enough dispatchable capacity (often gas-fired) is available in a country.
The first objective of the use of these support measures is to enable the deployment of technologies that would initially be too costly to build only based on market forces, allowing for the achievement of cost reductions thanks to economies of scale. The most successful example in recent years is given by solar PV. A second purpose of these measures is to provide additional revenues to low-carbon technologies (e.g. ZECs for nuclear50 for not emitting CO2 and other pollutants), and allowing them to offset lower wholesale prices. A third reason is to minimise uncertainty regarding future revenues, hence reducing risk and consequently the cost of capital, which is a major cost component for capital-intensive, low-carbon energy sources such as nuclear power, wind power or solar PV. As the cost of these support measures is most often included in the final-users’ bill, a major concern is the affordability of electricity for all consumers.
Nuclear power has a number of similarities to all other low-carbon technologies, and some elements that are peculiar on their own. The similarities include: the capital-intensive nature of the projects; the strong impact of the weighted average cost of capital (WACC) on the overall cost and the importance of reducing the risks associated with each project; the role of support policies for their deployment. The peculiarities include: the sheer size of the capacity (most reactors being in the range of 1.000 to 1.600 MW and being built in pairs), which results in very large overall investments; the long lead times to build the plant, which represent a major risk factor for overall cost increases following delays; the long lifetime, which assures reliable production for several decades, but is exposed to possible policy changes.
Lifetime extensions of existing power plants (provided that the necessary safety and security conditions are in place) are generally the cheapest way to provide dispatchable low-carbon electricity generation, together with some investments in renewable energy sources such as hydropower. The cost of lifetime extensions can be estimated in the 40-60$/MWh51 range, assuming an 8% cost of capital. Limiting the risks, both real and perceived, related to these investments can further reduce this cost estimate by lowering the cost of capital. On the other side, low wholesale prices, policy hurdles and the lack of long-term vision can hinder investments.
Decisive elements affecting the unit cost of new reactors are the type of model and location, the level of standardisation and the industrial organisation in the country where it is going to be built, as well as the regulatory regime. Additional costs and construction time overruns for some projects in Europe and North America have been sowing doubts about the economics of new reactors in these regions. These projects were the first ones to adopt a new technology (so-called first of a kind or FOAK), after a prolonged period of no new construction in the relevant countries. Significant cost reductions are expected to be achieved through the serial manufacturing of standardised plants52 and will be key to ensuring the future of their competitiveness with other technologies.
The current situation in advanced economies is in stark contrast with the cost reductions achieved for nuclear reactors built in the 1970s and 1980s, notably in countries such as France where a constant stream and standardisation of new plants allowed for an increase in the know-how of the industry and significantly reduced the investments costs (see figure on the next page). The cases of France and the United States differed mainly due to location and the standardisation of the reactors that were being built, highlighting how continuous construction of new reactors is fundamental to attaining cost reductions. In recent years, these cost reductions have been better mirrored (albeit to a lesser extent) in other countries – such as Russia and China – with several reactors being constructed, either in-house or abroad.
Overnight construction cost for nuclear plants in France and the United States
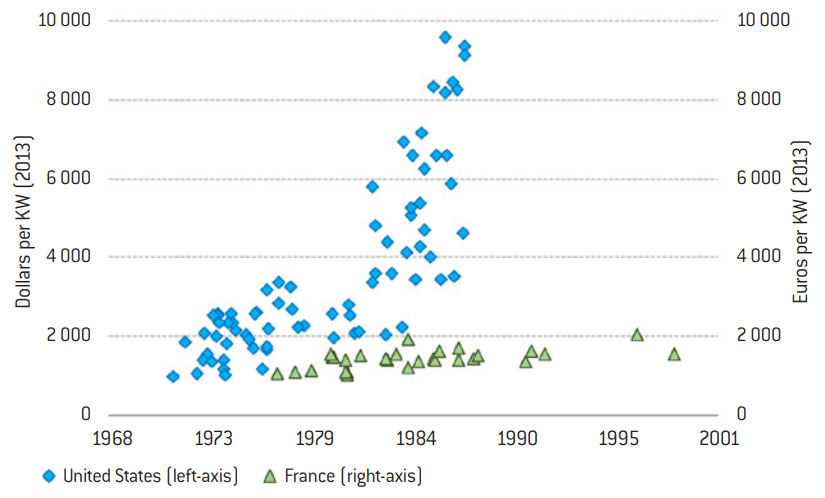
Source :
International Energy Agency (IEA), World Energy Outlook 2014, Paris, 2014.
Other energy uses
International Atomic Energy Agency (IAEA), Industrial Applications of Nuclear Energy, Vienna, 2017.
The use of nuclear energy is not limited to nuclear power. The decarbonisation of the energy system requires the decarbonisation of all its three major energy demand components: the power, heat and transport sectors. Nuclear power can provide an essential contribution to the decarbonisation of the power sector, but there are several other energy applications for which nuclear can be a cost-effective solution. These include district heating, industrial heat applications, hydrogen production, transportation and water desalination.
The use of nuclear energy for district heating, operating the plants in combined heat and power (CHP) mode, is not new. It has been used since the beginning of the exploitation of nuclear energy, for example in the United Kingdom, Sweden and Switzerland among advanced economies, and is still in use in Russia and parts of Central and Eastern Europe. This type of use can be particularly significant in remote locations, providing both power and heat, as in the case of the new SMR floating barge Akademik Lomonosov in Pevek, Russia.
In November 2019, China started to operate two new reactors at the Haiyang power plant in the Shandong province using Westinghouse’s AP-1000 technology to heat 700.000 square metres of housing. The country is also at advanced stages of development of two district heating reactor models (that produce only low-temperature heat and no power), for a total thermal power of 200 and 400 MWt respectively. These reactors are considered to be key low-carbon alternatives to coal-fired district heating in the north of the country, particularly for inland use.
Other countries have expressed a high interest for low temperature heat- only power reactors as a means of decarbonising the current district heating systems. An example is Finland, whose VTT Technical Research Centre announced in February 2020 the launch of a project to develop a SMR for district heating that could be used in several cities to replace the use of fossil- fuel based generation.
The use of nuclear energy for steam generation to be utilised in industrial applications depends on the type of utilisation, and on the temperature and pressure levels of the steam needed. The high working temperature range of certain reactors – such as the high temperature gas reactor (HGTR) SMR under construction in China – make them more suitable for some of these applications. The deployment of this type of reactor in industrial hubs to be used in CHP mode could prove to be very attractive53.
Hydrogen has the potential to play a crucial role in future low-carbon scenarios, as briefly discussed in section II.1. Hydrogen production cost hinges on several parameters, depending on the inclusion or not of CO2 pricing. With no CO2 pricing and at today’s gas prices, natural gas is the principal and cheapest source of hydrogen production, while nuclear energy can be cost competitive among low-carbon technologies. The choice of the type of nuclear reactor used for hydrogen production can also greatly vary depending on the type of hydrogen technology, with larger nuclear reactors to likely be used in CHP mode and SMRs to be solely dedicated to hydrogen production.
An additional use of nuclear energy is for water desalination, which is of particular interest in fresh water-scarce countries. An example of this is Saudi Arabia, which has signed a memorandum of understanding with South Korea for the first commercialisation of their SMART SMR, for the production of electricity and seawater desalination.
The use of nuclear energy for transportation has historically been almost exclusively for military use, where it was mostly adopted in submarines and aircraft carriers. A critical exception is for civil icebreakers, and especially for the Russian Arktika-class icebreakers. On the military side, it should be noted that while most countries that have nuclear power plants do not have nuclear deterrence devices, almost all countries that have nuclear deterrence devices also have nuclear power plants.
Geopolitical implications
European Commission (EC), Study on energy technology dependence, Brussels, 2019.
The use of nuclear technology has a panoply of geopolitical implications. These include the links created between the manufacturing company/country and the company/country where the plant is being built, the impact on energy resilience, on technology innovation, on medical uses and on energy security. The latter have been put under the magnifying lens even more so during the Covid-19 emergency, also highlighting how traditional concerns over oil and gas imports can progressively shift towards concerns over technologies and materials needed for decarbonisation54.
Manufacturing and technology export
Center for Strategic and International Studies (CSIS), The Changing Geopolitics of Nuclear Energy, 2020.
Westinghouse, “Westinghouse significantly expands fuel supply in Ukraine”, 2020.
United States Department of Energy, Restoring America’s Competitive Nuclear Advantage, 2020.
Author’s analysis following a cautious implementation of countries’ deployment plans, accounting for possible barriers and delays.
Construction of a second unit in Turkey started in April 2020.
State Atomic Energy Corporation Rosatom, Performance in 2018.
Center for Strategic and International Studies (CSIS), 2020, op.cit.
Nuclear manufacturing for domestic and international markets establishes strong geopolitical relationships and can have profound implications. Historically, the U.S.-born technology was transferred to France, Germany, Italy, Belgium, Sweden, Japan, South Korea and several other European countries, where the geopolitical coherence strong. Advanced economies’ nuclear reactors were then installed in Argentina, Brazil, China, India, Mexico, Pakistan, South Africa, and Taiwan.
The manufacturing of new power plants creates links over the several years in which the plant is being built, but also over the following years or decades, especially concerning the nuclear fuel cycle (fuel supply and spent fuel reprocessing) and potentially for maintenance support55. For newcomer countries, training of personnel can also be of importance. The reliance on imported nuclear fuel from one supplier can be compared to the import of fossil-fuels from one country only, although an amount of nuclear fuel equivalent to several years of operation can be stored easily. The importance of having more suppliers in the world can be exemplified with the case of Ukraine importing Westinghouse-produced nuclear fuel to be used in its Russian VVER nuclear power plants56.
The financing of new power plants is also a fundamental issue. New projects require very high investments, often in the order of several billion euros. Difficulties can arise in competitive markets without additional support, but investments in regulated markets can also prove to be challenging due to the large size of these projects. This can be a significant obstacle, particularly in emerging and developing countries where access to finance is more difficult, as in the case of South Africa which has been struggling to secure financing for its new nuclear projects for over a decade. Russian’s Rosatom and Chinese manufacturing companies often include favourable financing conditions, hence increasing the attractiveness of their projects. The U.S. International Development Finance Corporation (DFC) recently proposed to review the ban on the international financing of nuclear projects, mainly aimed at the possibility of offering financing for SMRs. The U.S. Department of Commerce estimates the nuclear reactor market will be worth $500-740 billion over the next ten years57.
From 2010 to 2019, the construction of 63 new reactors commenced worldwide (compared with 53 over the previous decade). Two-thirds of the international market adopted Russian and Chinese technology and this share is set to increase in future years following increases in Chinese manufacturing, the implementation of the agreements already made by Russia with several other countries, and the shrinking number of projects in advanced economies. Over the coming thirty years, more than 400 reactors (excluding SMR) are expected58 to start construction (see figure on page 50). Around 150 of these are in international markets (i.e. outside of the countries where the manufacturer is located).
Currently, China has the largest deployment plans while Russia is the largest exporter of nuclear power technology in the world. Russia currently accounts for over 60% of the global export market of new nuclear power plants; both to countries that already have the nuclear power technology and to newcomers – three out of four having opted for Russian’s VVER. Over the last ten years, two-thirds of the new global nuclear capacity commenced operations in China, both with domestic and imported technology. Russia, for its part, brought ten reactors online, has three reactors currently under construction and another four that have just started preparatory works for construction. It is building nine reactors in five other countries – Belarus (2), India (2), Bangladesh (2), Turkey (2)59 and Iran (1) – and four reactors are being built in Slovakia (2) and Ukraine (2) with a multi-contract approach. Russia also has further projects close to construction starts in China (4), Finland (1), Hungary (2), Egypt (2+2) and Uzbekistan (2); several others in different stages of planning60, including countries with plants already under construction; and ongoing discussions and agreements with the Czech Republic, Kazakhstan, Indonesia, Jordan, and Armenia. It is also competing for additional markets such as Saudi Arabia and South Africa.
China started the deployment of nuclear power relatively recently with French and Canadian technologies. Nuclear power only accounts for a small portion of the power mix in China. As of the late 2000s, the country started a programme that increased the installed capacity five-fold from 9 to 46 GW in just ten years. The development of domestic technologies and the firm intention to further develop the role of nuclear power in the electricity mix are positioning China as a key nuclear manufacturer for both domestic and export markets. Until now, China has only built new reactors abroad in Pakistan, using its CNP technology (Generation II), and currently has two Hualong One (Generation III) reactors under construction. The latter is China’s flagship technology, for which it has clear and evident high export ambitions. Current plans are to build one or more of these units in Argentina and two are under application review in the United Kingdom (where Chinese companies also have a 33.5% share in the Hinkley Point C project). China expects the Belt Road Initiative (BRI) to facilitate the adoption of this technology, with China’s nuclear industry expecting to be able to export over 10 units annually after 203061.
About two-thirds of the international (excluding the domestic) market from 2030-2050 remains open for competition (see figure on the next page). Traditional manufacturers in the United States, France, Canada, Japan, and South Korea are currently struggling to develop further projects, both domestically and internationally, often on the back of economic factors and a lack of policy support. However, developing projects in a continuous manner can provide substantial support to reduce costs. Over the last twenty years, construction was started for 17 reactors domestically and 13 reactors internationally with advanced economies’ nuclear technology. This is insufficient to ensure a strong cost reduction.
Start of construction of reactors by major manufacturers, for domestic and international markets, by decade between 2000 and 2050
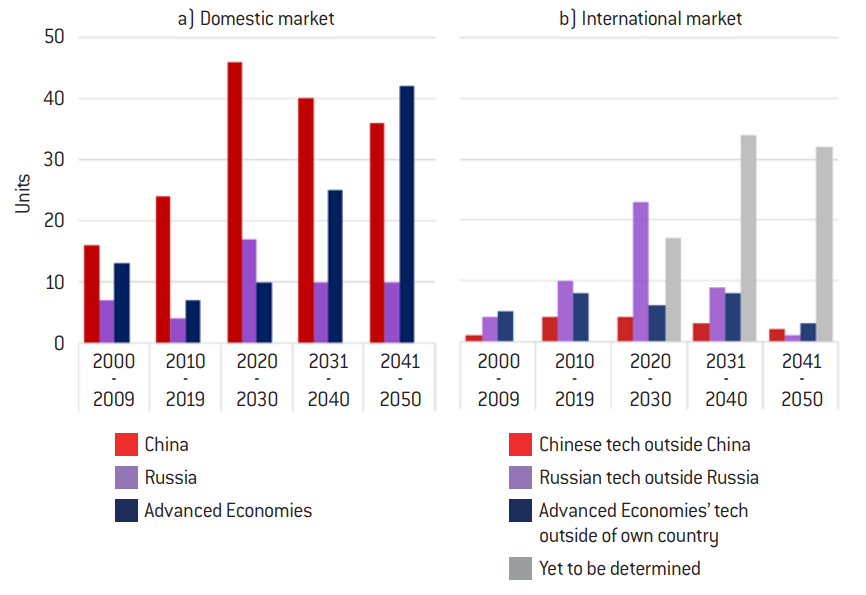
Source :
International Atomic Energy Agency (IAEA), Power Reactor Information System (PRIS) database, Vienna, 2020 for under construction and the author’s analysis for future evolution.
Note: SMRs are not included in this graph.
The 13 reactors started on the international markets were developed with Westinghouse’s AP-1000 (4 reactors in China), KEPCO’s APR-1400 (4 reactors in UAE) and Framatome’s EPR (1 reactor in Finland, 2 reactors in China and 2 reactors in the United Kingdom). The hurdles for further domestic deployment in the United States and South Korea can raise doubts on the ability to further export Westinghouse’s and KEPCO’s technology respectively. The replacements that will be needed as of the end of the 2020s/beginning of the 2030s represent a crucial opportunity to achieve these cost reductions, provided that detailed planning is put in place. A robust and continuous chain of new builds is key to maintaining the industry know-how – e.g. in Europe – leveraging on existing skills and providing the opportunity to reinforce the existing industry value-chain and assets, and to use their competitive edge.
Energy security, resilience and the impact of the Covid-19 crisis
International Energy Agency (IEA), Energy security page, Paris, July 2020.
International Energy Agency (IEA), “Electricity security, Vital to well-functioning modern societies and economies”, 29 October 2020.
European Commission (EC), 2019, op. cit.
Nuclear power is a key technology contributing to energy security, but to warrant energy supply, technology security must be assured as well. Energy security is defined as “the uninterrupted availability of energy sources at an affordable price”62. In the past, the key concerns were mainly associated with the dependency on fossil fuel imports (mainly oil and gas), the geopolitical origins of these energy sources and their prices. These preoccupations were of primary importance for the advanced economies that consumed the bulk of these sources. Nowadays, with little or no demand growth and increasing production from unconventional sources in these countries, oil and gas security concerns are shifting more towards emerging and developing countries – with China and India at the forefront – where most of the growth of demand for these fossil fuels is coming from.
Nuclear power contributed to the decrease in the dependence on these sources for power generation in several advanced economies over recent decades. For example, in France oil-fired electricity generation accounted for 40% of the total mix in 1973 (first oil shock), before the country implemented its strong nuclear energy deployment programme. The role of nuclear power in broader considerations of energy security and import dependencies are also subject to the sourcing of the entire fuel supply chain – uranium extraction, conversion and enrichment. As many countries have fuel stocks for a typical duration of two to three years, nuclear power is generally accounted for as an indigenous energy.
Energy security concerns have been including evermore electricity security ones. Over the last ten years, an Electricity Security Action Plan (ESAP) was endorsed by ministers from member countries of the International Energy Agency, focusing on the electricity security challenges during the transition to low-carbon energy systems63. With increasing generation from variable sources such as wind power and solar PV in the electricity systems, nuclear power is confirming its important role in low-carbon technology for maintaining grid stability and inertia and, when called upon, to provide flexibility to the system (see section II.1).
The push to use cleaner forms of energy and associated technologies has started to raise additional concerns about the sourcing of these technologies and the materials used to produce them, ultimately highlighting the risk of moving from a fossil-fuel dependency to a technology dependency. Where these technologies will be produced and how the fuel (if any) will be sourced is of primary geopolitical interest. These preoccupations can relate to many low-carbon technologies, including nuclear power (see section III.1), wind power, solar PV and battery storage technologies. A study by the European Commission64 looks at the critical dependencies of these last three technologies in detail, with policy recommendations impacting EU security of supply policies and EU industrial competitiveness policies.
The Covid-19 pandemic has had a strong impact on energy production and consumption, highlighting additional energy security issues and the need for resilient energy systems. Over recent months, the spread of the virus has dramatically changed the organisation of activities in our societies. This has also been the case in energy systems, with a significant drop in fossil-fuel demand and certain energy prices, such as in the case of the use of oil in the transport sector. The importance of energy security has been gaining even more prominence, stressing the importance of resilient systems. Electricity security has reaffirmed its key role in our societies, for the functioning of essential services such as hospitals, but also for enabling internet networks to support teleworking.
Most electricity systems have seen a significant drop in overall demand, with the corresponding generation characterised by three main trends: increasing generation from renewables (mainly wind and solar PV capacity installed in the later part of 2019), backing-off fossil fuel-fired generation (mostly coal) and reduced generation from nuclear in certain systems (such as in Europe, where nuclear power significantly contributed to the flexibility of European systems). This context resulted in a further increase in the share of wind and solar PV generation in the power mix compared to previous years. This situation, though, cannot fully be considered as a test of how future systems could look in more advanced stages of the energy transition due to the existing redundancy of capacity and flexibility present in the current systems, which are dimensioned for higher demand.
Whether this increase of wind and solar PV will represent a significant change and become more permanent, and what role nuclear power will play as a decarbonisation and flexibility option, depend on future investment in low- carbon technologies themselves and in other options. These investments could be reduced or delayed due to lower capital availability, decreased revenues of electricity companies (and even households investing in these projects) and the deferral of projects, or they could potentially increase if policy makers include them in the stimuli packages that are being considered in many countries.
Nuclear power plants demonstrated a great degree of resilience in light of the electricity demand changes following the Covid-19 pandemic, with continuous operations and no forced shutdowns of power plants. Nuclear power generation decreased in certain countries, with increasing flexibility following the changes in electricity demand and the needs of power systems, while ensuring the safety of the personnel, contributing to the stability of the grid and providing low-carbon generation. Investments in nuclear power can have a dual approach in any stimulus package, creating the right conditions for investments in lifetime extensions in the short-term, and providing support for investment in new plants in the long-term. Europe can still get a hold of this opportunity, despite leaving it unseized for now in the EU plan NextGenerationEU and postponing the decision for inclusion in the EU Taxonomy. The inclusion – or not – of nuclear power in the EU Taxonomy can play a fundamental role (see section I.2.b), though its consequences are not only limited to Europe. As this is the world’s first-ever classification, the decision could have significant repercussions in several other emerging and developing economies that could face more difficulties with some usual lending institutions. This could also represent a further push towards financing models such as those provided by Russian or Chinese companies.
Technology innovation
Stephen M. Goldberg and Robert Rosner, Nuclear Reactors: Generation to Generation, American Academy of Arts and Sciences, 2011.
Philippe Passebon, “Nucléaire : les robots et technologies de maintenance d’Areva récompensés par les WNE Awards”, Industrie & Technologies, 29 June 2016.
United States Nuclear Regulatory Commission (U.S.NRC), Backgrounder on High Burnup Spent Nuclear Fuel, 2020.
Nuclear power is a story of constant innovation that spans over many decades, and continues to be strong. Industry know-how should therefore be carefully evaluated, as well as the risks and costs of losing it. Design of nuclear reactors keeps evolving in terms of efficiency, safety and performance. This evolution can be broadly categorised in Generation I (prototypes and early movers, none of which are online today), Generation II (accounting for almost 95% of the reactors currently online, with a typical initial lifetime of 35-40 years, and the possibility to extend to 60, and, in some cases, to 80 years), and Generation III (the majority of plants under construction today, with enhanced active and passive safety measures that can contain major accidents and attacks with minimal or no impact outside of the power plant itself, a typical lifetime of 60 years and the possibility to extend it to 80 years and possibly beyond)65. It includes a large variety of design and types, using diverse technologies, fuel assembly, moderators and coolants.
This variety has seen a progressive convergence towards the LWR (Light Water Reactor) type, which now accounts for 90% of reactors currently under construction, and notably for the PWR (Pressurised Water Reactor) type.
The current research and development look at a number of new designs, following four main streams: small modular reactors (SMR – see section III.3.a), Generation IV reactors, nuclear fusion and experimental reactors (including those to produce medical radioisotopes). The distinction between these groups is not always clear cut, with some SMRs being of the Generation IV type.
In addition to R&D for new reactors, critical innovation steps are being taken for enhancing flexibility of current and future power plants, improving maintenance and reducing costs through digitalisation, creating “numerical twins”, using robots66, and improving the efficiency of fuel use, requiring less and less fuel for producing the same kilowatt-hour (kWh) of electricity67.
A significant portion of this innovation is being conducted in advanced economies, thanks to the industry and university knowledge, as well as experience cumulated over the past decades. In particular, the United States and Canada are especially active on the SMR front, the largest project in the world on nuclear fusion is being built in the south of France, and many laboratories and research centres are exploring new advancements in these areas. Nonetheless, the scientific research, know-how and innovation is gradually shifting outside of advanced economies. This situation is not just limited to nuclear power, but is being seen, for example, in solar PV (with the majority of manufacturing located in China and Taiwan) and in batteries.
a) Small modular reactors (SMR)
Small modular reactors (SMR) are aiming at four goals: reduced costs, increased security, adapting to the increased flexibility needs of power systems, and spreading to new markets. The history of nuclear reactors has seen the increase of reactor size from an average of a mere 136 MW of the Generation I type to a typical size of 1.000-1.600 MW for the Generation III (although some smaller versions exist), mainly in the quest for increasing security and decreasing the cost per unit of power (see figure on the next page).
SMRs are defined as reactors with an installed capacity smaller than 300 MW, with most versions in the 50-200 MW range (see table on page 56). A subset of this category is represented by micro modular reactors (MMR), with an installed capacity smaller than 10 MW.
As seen in section II.3, one of the difficulties of achieving cost reductions for larger projects is being able to have a continuous series of new reactors being built in order to profit from industry know-how and experience. The aim of SMRs is to reduce costs not through increasing size, but rather through economies of scale by producing many of the same type of units that are identical to one another.
Global average size of reactors and start of construction by decade – 1950-2019
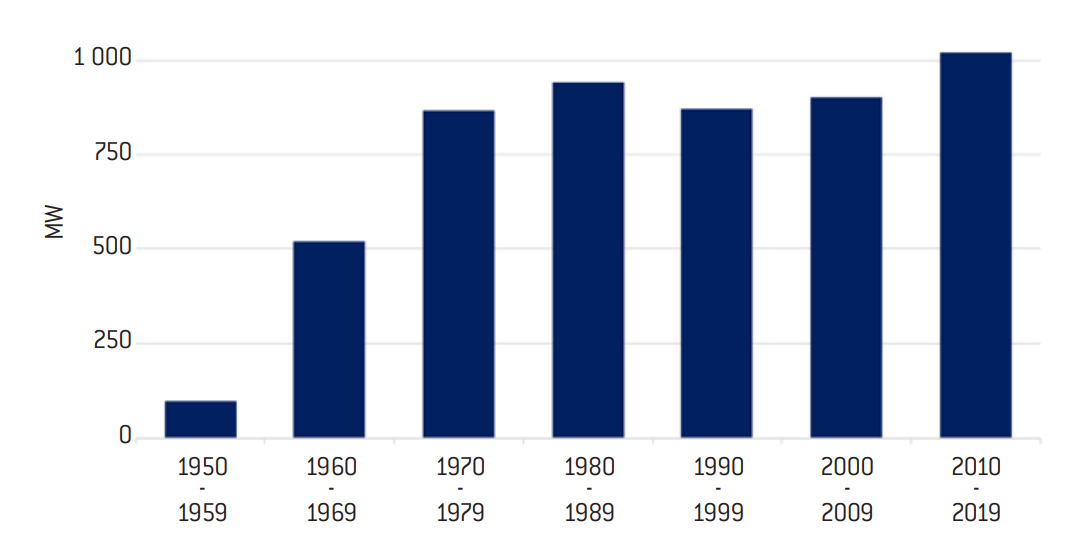
Copyright :
© Fondation pour l’innovation politique – December 2020
Source :
International Atomic Energy Agency (IAEA), Power Reactor Information System (PRIS) database, Vienna, 2020.
Having smaller sizes of reactors would also have a number of advantages, including much smaller investment requirements and shorter lead times, with both factors contributing to facilitating a simpler financing of projects. As several of these projects (e.g. NuScale in the United States or the HTGR reactor in China) foresee the deployment in each site of 10-12 units, the economies of scale are expected to compensate the diseconomies of size. Furthermore, SMRs can contribute to the flexibility of power systems thanks to their modularity. This characteristic can be particularly attractive in smaller power systems, eventually opening new markets that are currently unsuitable to the standard large Generation III designs.
Main SMR projects and related manufacturers and countries
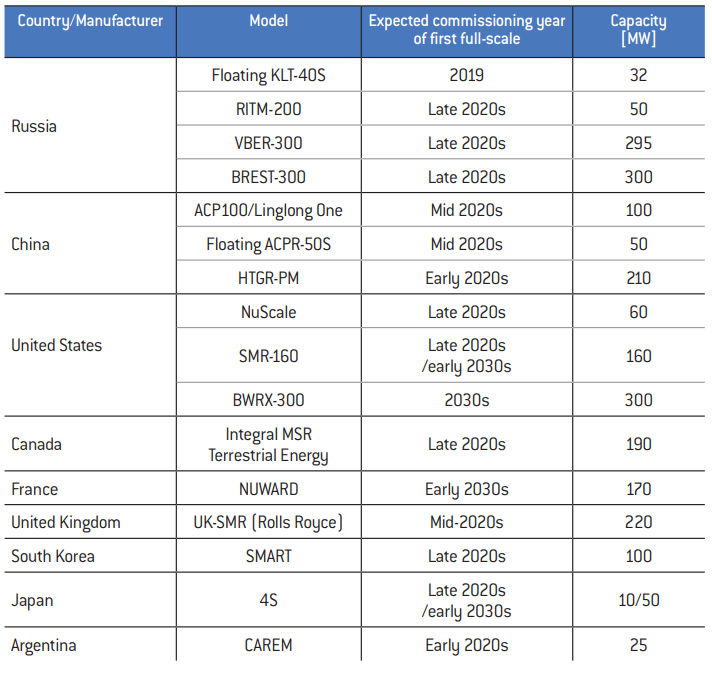
Source :
International Energy Agency (IEA), Nuclear Power in a Clean Energy System, 2019, op. cit.; International Atomic Energy Agency (IAEA), Advances in Small Modular Reactor Technology Developments, Vienna, 2018; International Atomic Energy Agency (IAEA), Advanced Reactor Information System database, Vienna, 2020.
Nuclear Energy Agency NEA/OECD, 2019 NEA Annual Report, Paris, 2019.
Canada SMR Roadmap, 2018.
World Nuclear Association (WNA), Generation IV Nuclear Reactors; International Atomic Energy Agency (IAEA), Advanced Reactor Information System database, 2020, op. cit.
International Thermonuclear Experimental Reactor (ITER), “What is ITER?”, 2020.
The commercial deployment of these type of reactors is not expected before the second half of the 2020s at the earliest. Necessary conditions to their success will be the ability to deliver the expected cost reductions and to reach full standardisation. In this respect, strong effort is being put in place in the United States and Canada, with significant levels of R&D spending68 and agreements towards common standardisation69. Several other countries are exploring the SMR option: China is currently building a prototype of HTGR, expected to come online next year, and is planning to start the construction of two ACP100 next year; Russia brought online a floating barge with two nuclear units for use in remote areas of the country last year; Argentina is building a prototype; South Korea (with an agreement with Saudi Arabia), Japan, France and several others are also exploring additional designs. The deployment and level of competitiveness of SMRs could change the deployment of nuclear power and the equilibriums across countries depending on when they will be fully available at scale and cost.
a) Generation IV
Research and development continues to support the quest for safer, more efficient, more economical and universal nuclear energy. The next generation of nuclear reactors (so-called Generation IV) is aiming at further enhancing safety, minimising waste (both in terms of quantity and in terms of duration), being proliferation-resistant by design and able to provide solutions for hydrogen production, industrial heat and water desalination. Six types have been identified, most of them with closed fuel cycles able to minimise high-level waste70. Some of these projects are in very well-advanced state of research, while others remain at the concept level. Overall, the Generation IV reactors include:
- Gas-cooled fast reactors (GFR)
- Lead-cooled fast reactors (LFR), with projects in Russia and Belgium
- Molten salt reactors (MSR)
- Sodium-cooled fast reactors (SFR), under construction in Russia (1), China (1) and India (1)
- Supercritical water-cooled reactors (SCWR)
- Very high-temperature gas-cooled reactors (VHTGR), with one prototype under construction in China
b) Nuclear fusion
Further research is being conducted on the nuclear fusion front, albeit with a longer time frame. Among the several projects being explored, ITER (International Thermonuclear Experimental Reactor) is the largest project in the world for nuclear fusion research71. It is currently being built at Cadarache in the south of France, funded and based on an international collaboration between the European Union, the United States, Japan, South Korea, China, Russia and India.
Started in 2013 and expected to come online around 2025, ITER will be a 500 MW tokamak fusion device (requiring an input of around 10% of its output power) designed to prove the feasibility of nuclear fusion (a phenomenon similar to what happens in the sun). With very limited nuclear waste and no risk of meltdown due to the intrinsic nature of the technology, nuclear fusion holds great promises, though a commercial reactor is still several decades away.
Medical radioisotopes
International Atomic Energy Agency (IAEA), Research Reactor Database, Vienna, 2020.
Molybdenum 99 is one of the main medical isotopes and is widely used to diagnose heart diseases and cancer, as well as for other important medical applications.
Nuclear Energy Agency NEA/OECD, The Supply of Medical Radioisotopes, Paris, 2018.
A key aspect of nuclear know-how is linked to the production of radioisotopes for medical use. These are primarily used for two purposes: diagnostic applications (with millions of exams in Europe annually) and therapeutic applications (mainly linked to cancer treatments). Given the radioisotopes’ short lifetime, their production needs to be continuous. Medical radioisotopes can mainly be produced in cyclotrons or in nuclear reactors. There are about 1.200 active cyclotrons in the world, 200 of which in Europe and about 20 in France alone. The first five actors account for about 90% of global production. There are about 220 nuclear research reactors in the world72, including those used for the production of isotopes. Primarily, there are two types: irradiation reactors (fewer and more complex) and neutron-source reactors (less efficient, but simpler).
The irradiation reactors are the central source for the production of molybdenum 9973. After the closure of Osiris in France in 2015 and NRU (National Research Universal reactor) in Canada in 2018 (respectively third and first largest experimental reactors in the world), there are now 10 reactors worldwide that assure the molybdenum production. Five of these are in Europe (in Belgium, the Netherlands, Poland, Germany and the Czech Republic), accounting for over half of global production74.
Similar to the situation experienced by power reactors, experimental reactors are also ageing rapidly. In 2016, about 90% of global molybdenum production was met by seven reactors, five of which are in Europe and four of which are over 45 years-old today (see table on the next page). Several shortages have been experienced over the last decade, principally due to reactors’ outages. In the short-term, to avoid such shortages, better coordination at the European and global level has been put in place. However, replacements will be needed soon. By around 2025, three new reactors are planned, yet they have been facing delays and investment uncertainties.
The nuclear industrial know-how is critical for nuclear power, medical supplies, as well as several other uses. R&D, innovation and investments in new assets will be critical to maintaining and expanding this knowledge.
Main Experimental Reactors in Europe for molybdenum production
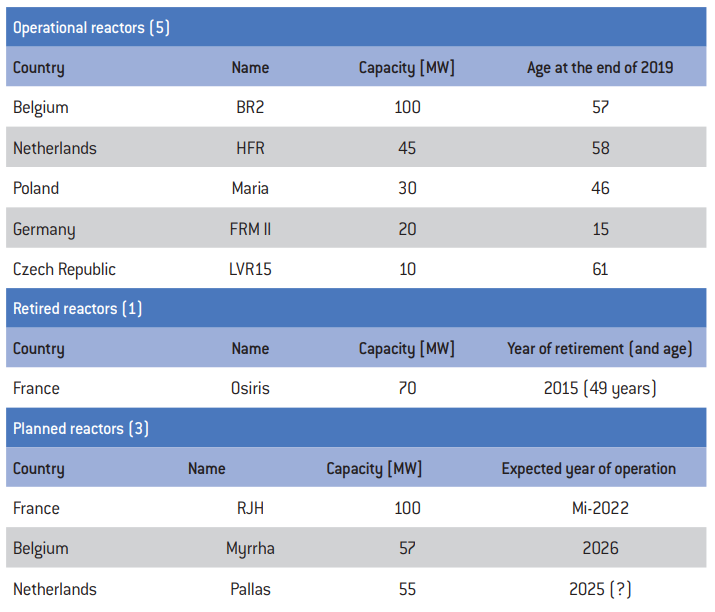
Copyright :
© Fondation pour l’innovation politique – December 2020
Source :
Nuclear Energy Agency NEA/OECD, The Supply of Medical Radioisotopes, Paris, 2018.
Climate change is becoming an ever-urgent issue, requiring unprecedented action. The Paris Agreement of 2015 commits to limiting the increase of global temperature to well-below 2 degrees Celsius and efforts to keep it at 1.5 degrees. In order to achieve this goal, all low-carbon options are necessary: energy efficiency, renewable energy sources, nuclear energy, carbon capture, utilisation and storage (CCUS). The mix and deployment of these technology options is set to change according to potentials and constraints in each region. Yet, some countries have decided to dismiss certain proven technologies (such as nuclear power and large hydropower) or emerging ones (such as CCUS). While the choice of available options is an evident prerogative of each country, questions arise concerning the increase of costs due to their restriction and the ability to decarbonise in the short available time, as well as to where science-led policy ends and ideology begins.
The decarbonisation of the power sector is a decisive step towards the decarbonisation of the overall energy sector, but it is not enough. Reaching the goals set in the Paris agreement requires the decarbonisation of all end-user sectors within just a few decades. Three paramount levers to achieve it are: the electrification of end-uses, the decarbonisation of heat demand and the wider penetration of hydrogen in the energy mix. As a consequence, electricity demand could more than double over the upcoming decades. Nuclear energy can play an important role in each one of these three areas, the most predominant one being in the power sector as a low-carbon source, as a source of flexibility and contributing to electricity security of supply. Nuclear energy requires a number of conditions to provide a successful contribution to these goals. Addressing public concern is essential, with proactive, transparent and independent nuclear safety regulators. We must learn from past successes and failures, establish clear roadmaps to provide long- term visibility to all actors, and allow cost reductions through the continuous building of plants. It will also be essential that we pursue the standardisation of design while meeting the highest levels of safety standards, identify the most effective market design reforms while preserving technological competition, and maintain a good industrial ecosystem while ensuring scientific know-how and innovation.
Europe is aiming to be the first carbon neutral continent by 2050, yet its energy policy lacks coherence and is often unclear on nuclear power. On the one hand, nuclear power is the largest low-carbon power-generating source today, and is included in the EU’s future scenarios as an essential low-carbon technology.
On the other hand, the European Union struggles to include it in the Taxonomy as a key option for a decarbonised future. Postponing this decision can strongly impact its effectiveness and put the ability to reach its climate goals at risk.
Europe has a strong nuclear industry and know-how, and a long tradition of nuclear energy innovation. Yet, China and Russia are taking the lead on nuclear development, India has strong nuclear power ambitions, and the United States and Canada are pushing on SMRs. Europe risks losing its competitive advantage without a clear roadmap in France and with several Eastern European countries that might ultimately turn towards other suppliers in the absence of a robust and cohesive European nuclear energy policy. The economic, industrial, and geopolitical consequences of inaction are strong, and even delaying decisions can endanger the economic and energy sovereignty of Europe. The message is therefore clear: Europe needs to act, immediately.
The author wishes to thank Michel Berthélemy, Jean-Paul Bouttes, Albert Bressand, Marco Cometto, François Dassa, Dan Dorner, Henri Paillere, John Parsons, Brent Wanner and David Wilkinson for their comments, views and support. The views and opinions expressed are solely the views of the author and do not represent a statement of the views of any other person or entity.
No comments.